7 Earth’s Natural Hazards
Jason Kelsey
The living and non-living systems we studied in Part Two are regularly subjected to stress. As we will see in detail in Part Three, although much of this pressure comes from human activity, powerful and disruptive natural phenomena also can bring about substantial changes to landforms, waterways, organisms, and ecosystems. The bottom-line message is: Earth has always been a risky place to live. Even before people and their technology started to spread across the planet, members of the biosphere were in near constant danger. In this chapter, we will briefly consider the origins and consequences of some of those natural hazards.
Key concepts
After reading Chapter 7, you should understand the following:
- How anthropogenic and natural hazards differ and why this distinction is important to environmental scientists
- The causes (review Chapter 3), consequences, and relevance of earthquakes and volcanic eruptions
- The many natural and anthropogenic phenomena that contribute to mass wasting
- How floods occur, can be predicted, and how human land-use decisions can make them more destructive
- How human activity may worsen the effects of biological stressors like pathogenic bacteria
7.1. CLARIFYING THE MEANING OF OUR TERMS
Stressors are assigned to one of two groups: those that are brought about by humans are said to be anthropogenic, and those that occur in the absence of humans are natural. This scheme is not perfect, and it might even induce an argument about the meaning of the word “natural” and how it applies to humans. However, it is a useful approach because it allows us to distinguish between hazards we can control and regulate and those we cannot. Any lingering values-based questions about our terminology will need to be addressed in venues more appropriate for such discussions than this book.
7.2. PHYSICAL STRESSORS
Here we take a quick look at some non-biological forces that shape the Earth and its organisms. Note that our coverage of these subjects is not meant to be exhaustive but rather is intended to provide an overview of and some background about important stressors and the effects they can induce. Many detailed sources of information about these topics are available, and you are encouraged to consult them for additional information (start with the web link provided at the end of this chapter).
7.2.1. Earthquakes: the first of two hazards caused by moving tectonic plates
Vertical shaking
Earthquakes are vibrations of the surface caused by movement along faults. A fault is often visualized as a crack in the crust, although it really is a surface, or plane, along which rocks can move relative to each other (Figure 7.1).
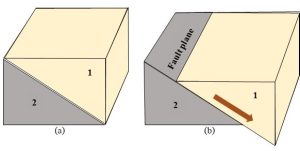
What causes faults to form? Recall that lithospheric plates are constantly in motion (Chapter 3). Movement in the vicinity of divergent, convergent, and transform boundaries applies gradual and persistent stress to the crust. Importantly, though, the rocks in such zones do not move constantly; instead, they absorb some energy while remaining stationary. When the accumulated stress eventually overcomes the strength of the materials holding the rocks together, sudden slippage occurs (known as rock strain). The amount of movement can range from nearly imperceptible to many meters in seconds or minutes. By and large, the severity of vertical shaking felt on the surface near a fault is proportional to the distance of displacement along that fault.
Effects
Damage at the surface. Vibrations have the potential to disrupt, damage, or destroy anything on the surface. We might be most familiar with the ways earthquakes affect buildings, roads, and other human structures (Figure 7.2), but they can also influence natural ecosystems. For example, trees growing on a slope could lose their support and fall over during an earthquake; as we know, secondary succession would likely follow such an event (Chapter 5).
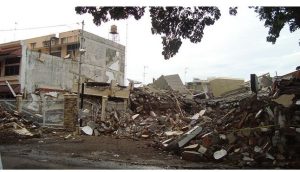
Landslides. As is described in the section on mass wasting (see below, after the section on volcanoes) earthquakes can trigger these important events.
Tsunamis. These are ocean waves induced by the sudden displacement of a large amount of water. They can be brought on by many forces, including a large asteroid or piece of continental crust splashing into the ocean or even localized high winds. An earthquake that occurs on the ocean floor can also start the process of tsunami formation. In short, movement along a submarine fault can cause water to quickly shift position and form a temporary bulge on the surface. It is worth noting that in the open ocean, the wave created by an earthquake is very difficult to detect—even passengers in a boat directly above a fault would likely be unaware of what is happening beneath them. However, as the bulge moves toward coastlines it changes from a subtle, horizontally oriented wave, to a predominantly vertical wall of water. By the time it strikes the beach, it can range from a few centimeters to 10 meters in height (Figure 7.3).
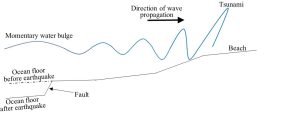
Clearly, a very tall tsunami could be extremely destructive, affecting both human and natural systems. In 2004, for example, a powerful earthquake in the Indian Ocean led to a tsunami large enough to kill well over 200,000 people as well as pummel coastal ecosystems (Figure 7.4). See Box 7.1 for more about tsunamis, including how humans can detect and respond to them.
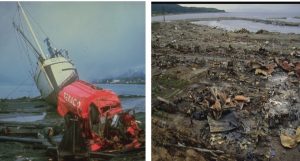
Box 7.1. Tsunamis: fascinating and deadly
Contrary to what you might expect, people often walk toward instead of away from the beach in the moments before a tsunami occurs. What accounts for this seemingly illogical behavior? In short, tsunamis are preceded by very rare and interesting phenomena that few people recognize as a warning. It starts when water suddenly and dramatically moves off the beach, straight out to sea. As a result, fish and other tasty organisms that ordinarily live under water in that zone are revealed. Many observers cannot resist the temptation to scoop up the fresh seafood just lying in the wet sand, so they walk into danger instead of running inland as fast as they can toward higher ground. Unfortunately, the propagating waves quickly bring back all of that missing water—plus much more—to the coastline in a potentially tall and deadly wave. Governments and scientists alike are working to minimize the numbers of deaths by improving tsunami prediction, preparedness, and awareness. It has been difficult to achieve these goals, though. Since tsunamis are difficult to spot in the open ocean, we often do not know where and when they will strike until the last minute. Technologically developed countries have employed specialized buoys off their coastlines that are sensitive enough to detect the signs of impending tsunamis. The United States, for example, has an array of warning buoys deployed in the Pacific Ocean. In case of emergency, notification and evacuation plans are in place. A public awareness campaign has been launched as well, with signs such as the one in Figure 7.5 posted in risky areas. The level of preparation in developing countries, though, is inconsistent and often insufficient.
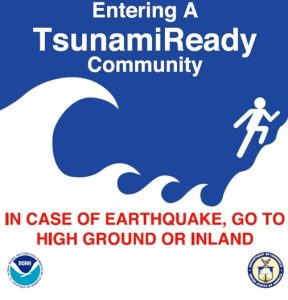
Susceptible locations
By studying plate tectonics and historical records, geologists have been able to identify regions most susceptible to earthquakes. Generally, they occur at or near plate boundaries (review Figure 3.3 in Chapter 3). So, places like southern California, U.S.A., Iran, and Tibet, experience more earthquakes than, say, those in central North America, southern Australia, or central Africa.
Prediction
Given the relationship between earthquake occurrence and tectonic plate boundaries noted in the previous paragraph, it is fair to say we know where they are likely to occur. However, it is extremely difficult to predict when they will strike a particular location. Geologists use two strategies to try to evaluate the risk for future earthquakes.
Historical data and long-term predictions. An evaluation of previous earthquake activity combined with knowledge of the properties of the rocks in a region can allow us to calculate the likelihood that an earthquake of a given magnitude will occur within a defined time frame. Unfortunately, the best we typically can do is narrow it down to years or even decades. Information provided is still useful, though, because it allows people to make long-term plans and modifications to vulnerable structures.
Precursor phenomena and short-term predictions. Earthquakes can occur without any notice, bringing about massive death and destruction to unsuspecting people. Sometimes, though, earthquakes are preceded by warning signs evident just before they occur (minutes, hours or days). These precursor phenomena include visible changes to the surface in an existing fault zone, sudden production of radon gas near a fault, abnormal behavior of waves artificially sent by geologists into a fault, and even changes to animal behavior (although that last sign is not universally embraced as valid or reliable). If detected and properly interpreted, human communities can make life-saving preparations.
7.2.2. Volcanic eruptions: the second of our two tectonic hazards
Explosions
Volcanoes are vents from which molten and solid rock, ash, and gases from the interior of the Earth are ejected to the surface and into the atmosphere. They vary in size and shape and occur both above and below sea level. Figure 7.6 shows two common types of volcanoes, relatively explosive cones (also called stratovolcanoes) and shields, from which lava tends to flow more gently. The details are unnecessary for us, but you should note that differences in chemistry of the molten material, which depends on the type of plate boundary involved, determine the shape and behavior of the resulting volcanoes.
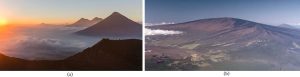
Effects
Volcanic eruptions can bring on many consequences to human and environmental health.
Death of organisms. Volcanoes are somewhat famous for their lethality. Among the many mechanisms by which they can kill people and other organisms is through molten rock (temperatures can reach 1000 °C or higher). Few common materials will resist incineration when they encounter lava. So, a volcano could destroy a large climax forest, leaving behind a barren landscape ripe for primary succession (Chapter 5). But it doesn’t stop there: their high-energy explosions can destroy nearby structures, and the large amounts of ash (essentially, tiny shards of glass that can injure lung tissues), and gases they emit can be deadly[1]. In other words, even if it does not bring on a fiery death, a volcano has other strategies it can use to kill you.
Change of climate. The tons of fine ash and aerosols ejected during some eruptions can disperse throughout the atmosphere and partially block incoming solar radiation (Figure 7.7).
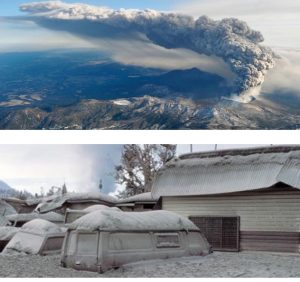
As a result, average global temperature may decline after a large eruption. The eruption of Mount Pinatubo in the Philippines in 1991 is one example of this phenomenon: measurable cooling of the entire planet occurred between 1991 – 1993. We will see more about the many factors influencing Earth’s climate in Chapter 14. Ash can also directly affect human civilization. Major eruptions in Iceland in 2010 halted air travel in Europe and North America because of the dangers posed by the materials they released into the atmosphere.
Contributions to acid precipitation formation. Some of the chemical compounds released through volcanoes can be transformed into acids in the atmosphere. So, following an eruption, both sulfuric and hydrochloric acids can be produced and change the chemistry of water falling to the surface. Acid precipitation can also be caused by anthropogenic activities, a topic we will return to in Chapter 14.
Susceptible locations
Volcanoes are usually found near divergent and certain convergent (in subduction zones) tectonic plate boundaries. For example, the large rim of the Pacific Ocean including Alaska, Japan, Indonesia, and Peru is sometimes called the “Ring of Fire” because it is defined by multiple plate edges and is the location of concentrated volcanic (as well as earthquake) activity (Figure 7.8).
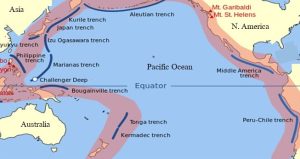
Volcanoes in places such as Iceland, Italy, and Tanzania also result from interactions among plates. In rare but notable cases such as the Hawaiian Islands, they occur far from plate boundaries.
Prediction
Volcanic eruptions have the potential to cause wide-spread death and destruction, so we are keenly interested in predicting and preparing for them. Like we see for earthquakes, both long-and short-term predictions are possible. Their locations near relevant plate boundaries, as well as their generally prominent appearance (e.g., a large, cone-shaped mountain) facilitate our ability to identify volcano-prone regions. In the near term, a volcano will provide many clues that an explosion is imminent, allowing for appropriate evacuations and other measures. Geologists (specifically, people known as volcanologists) study the sites of past activity and monitor gas emissions, changes in the shape of the surface, and other properties to assess the risk of impending eruptions.
7.2.3. Mass wasting
Downhill transport
Defined succinctly, this term refers to the gravity-driven movement of Earth’s materials from high to low elevation. Multiple variables are used to distinguish among the many specific types of phenomena grouped together here, including the rate of movement (ranging from nearly instantaneous to very slow), the role of water (liquid and frozen), and how dramatically the materials change form during their transport. So, for example, a landslide involves the rapid movement of relatively dry and intact rocks and soils down a sloped surface, whereas during a fall, rocks are not in contact with the slope as they move downward. Creep, as the name vividly suggests, is the very slow, all but imperceptible movement of generally intact soils, rocks, and even the plants growing on them along a slope. Figure 7.9 provides a simplified diagram of these three movements.
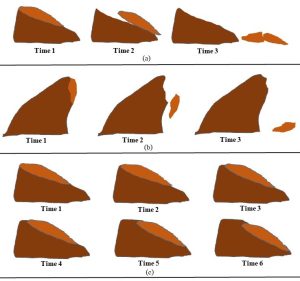
Note that several other types of mass wasting phenomena are possible, driven by different environmental conditions and affected by the properties of the rocks and soils present.
More on factors affecting mass wasting
Mass wasting occurs due to gravity—everything is pulled downward by this relentless force. Several other factors also play roles, influencing how readily materials will move downhill.
Presence and form of water. Liquid water can act as a lubricant and facilitate mass movements. So, a large and intense rainfall event can trigger a mud- or landslide. An icy layer under loose soil, common in polar regions, can also increase the likelihood of downward movement.
Presence of vegetation. Plants can help keep soil intact and therefore stabilize a slope for two reasons. First, the network created by their roots holds soil in place against the forces that would remove it (e.g., weathering by wind and water—see Chapter 3). We will return to this topic when we encounter soil erosion in Chapter 9. Second, their above-ground tissues like leaves block incoming rainfall, protecting the soil beneath from direct impact. As a result, the soil tends to stay in place. Natural fire and wind that remove vegetation, as well as human activities such as land clearing for farms (Chapter 9) and logging (Chapter 12) can bring on mass wasting. If the change occurs rapidly, as when an entire stand of trees is removed at one time (i.e., clear cutting), the risk of movement increases.
Steepness. A gentle hill will be less susceptible to mass wasting than will a cliff or steep slope. Natural erosion and human forces such as construction of roads and buildings can change the shape and angle of a slope (Figure 7.10).
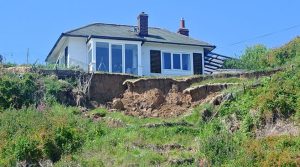
Triggering event. An unstable slope might maintain its integrity for an extended period. However, the vibrations of an earthquake might be just enough to dislodge the materials and send them downhill.
Susceptible locations
Mass wasting occurs anywhere there is a difference in elevation and gravity is active. In other words, it is not restricted to plate boundaries like we saw for the tectonic stressors—it can shape ecosystems all over the world. Now, it is important to realize that the specific type of event that occurs varies from place to place as a function of prevailing conditions. Movements in polar regions, for instance, are often affected by the presence of ice. Those in tropical regions are likely hastened by flowing water whereas desert landslides occur on slopes populated by minimal vegetation.
Effects
As we saw for the tectonic hazards above, both natural and human systems are affected by mass wasting. A common ecological consequence is seen in trees that develop curved trunks when they try to grow on a slope subject to creep. As their roots move downhill, they compensate by curling their crowns (i.e., their tops) toward the sun (Figure 7.11).
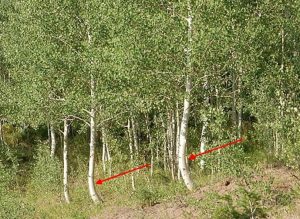
In fact, the presence of J-shaped trees on the side of a hill provides a clue that the slope is not as stable as it might appear. Eventually, gravity always wins the battle, and a climax community will be damaged or destroyed, making way for secondary succession. Death and destruction of human communities can also be the result of rapid events such as a sudden landslide or fall (Figure 7.12).
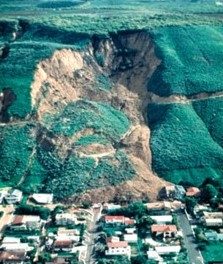
Prediction and prevention
An examination of the properties of the rocks and soils in an area as well as factors like slope angle, climate, and land use by people can help geologists calculate the risk of mass wasting in a location. Unlike earthquakes and volcanic eruptions, humans also have some degree of control over the occurrence of mass wasting. Clearly we cannot change gravity or weather, but we do have the ability to minimize activities that reduce slope stability (land clearing and poor construction choices, as noted previously). Alternatively, humans often attempt to minimize mass wasting after a poor land-use decision has been made. Retaining walls are one strategy used to slow the onset or effects of a landslide after, say, a road has been built on the side of an unstable hill. Unfortunately, they do not always work as hoped (Figure 7.13).
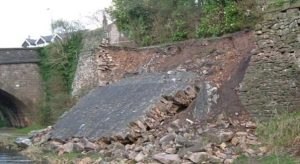
7.2.4. Extreme weather
Both human and natural systems are affected by the normal climatic conditions of their environments, which can include large seasonal fluctuations in temperature, moisture, and other conditions. As we know, organisms must adapt to these and other properties if they are to survive. However, relatively rare events such as hurricanes, thunderstorms, tornadoes, heat waves, and droughts can be sources of severe stress capable of damaging or destroying existing communities. Consider, for instance, that high winds associated with any of these phenomena could bring down trees, potentially setting the stage for secondary succession in a climax forest (Chapter 5).
7.2.5. Water-related hazards
Water is a powerful agent of change that can affect living and non-living systems in two distinct ways.
Weathering and erosion
As we learned in Chapter 3, water plays very important roles in the shaping of Earth’s surface. First, it is instrumental in the weathering of rocks and soils. Second, water can contribute to erosion, carrying away the products of weathering. These two processes can be accelerated when humans disrupt and destabilize soil, for example through agriculture (Chapter 9) and logging (Chapter 12). Although weathering and erosion do bring on some negative consequences in those cases, they generally do not create immediate hazards. The rapid removal of sand and soil beneath buildings and bridges, however, can lead to loss of both life and property. Structures on beaches and barrier islands are particularly vulnerable because those sandy features are inherently unstable. Day-to-day wind and waves along with periodic intense storms act to change the shape of coastlines and the human communities built there. In fact, hurricanes and similar events can remove entire beaches in a matter of days. Natural ecosystems in affected areas also can undergo dramatic transformations, stimulating succession and recovery.
Flooding
Input vs. output. There is not much mystery here: water will accumulate in a system if it enters faster than it is removed (Chapter 2). At some point, a reservoir’s capacity will be exceeded, and surrounding areas that are normally dry will be inundated. Consult Chapter 4 for more about pathways and reservoirs of water.
Effects.
Death of terrestrial organisms
Ecosystems characterized by predominantly dry conditions can undergo severe changes if water remains in them for an extended period. The large trees in a climax forest, for example, will likely die if they sit under water following a flood. These stands of dead trees will ultimately be replaced by secondary succession. See Figure 7.14.
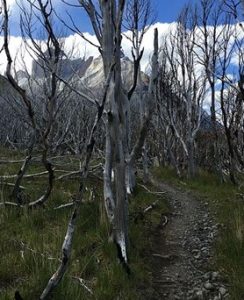
Spread of water-borne diseases
Certain pathogenic bacteria and protozoa (Chapters 3) from polluted waters can mix into human drinking water after flooding. Somewhat ironically, one of the outcomes of flooding is a profound lack of useable drinking water. If standing water persists for several days, people living in affected areas are at risk of contracting cholera and other diseases (Figure 7.15).
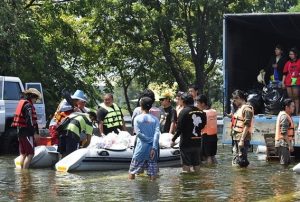
Property damage
Although clearly not an ecological concern, flooding can lead to massive loss of housing and other buildings as well as damage to crop and other valued lands.
Susceptible locations. Many factors influence the likelihood of flooding in an area.
Topography and climate
Perhaps the most obviously relevant factors affecting flooding are elevation, proximity to surface reservoirs, and amount of precipitation received. Low-lying homes near a river or ocean in areas subject to heavy rainfall are more likely to suffer flood damage than those on the tops of mountains in dry areas.
Surface properties
In addition to the important factors noted in the previous paragraph, severity and frequency of flooding is influenced by the types of soils and rocks present in an area. Permeability to water varies enormously among materials, so even if topography, climate, and other environmental factors are equal, the likelihood that rain will lead to a flood could still differ in two locations. Why? Recall from Chapter 4 that precipitation can experience two fates, infiltration (vertical downward movement) and runoff (essentially horizontal movement across the surface). Remember also that the relative importance of these two pathways is inversely related, meaning as one carries more water, the other carries less. If water cannot penetrate the surface (for example, if it encounters solid rock or tightly packed clay), a larger percentage of it will flow horizontally than vertically. Overland flow (i.e., runoff) tends to be more direct and efficient than is percolation underground, so if it dominates, nearby waterways will flood more readily than if infiltration is comparatively important. How much water gets to a reservoir within a given period is critical. If a fixed volume moves quickly to a stream, reaching its destination essentially all at once, flooding is more likely than if the arrival of that same volume is spread out over more time (again, using systems analysis, when rates of input exceed rates of output, flooding is the result). Both natural and human activities (described below) affect surface permeability and the speed of water movement downhill.
Vegetation present
Put simply, plants tend to slow the rate of runoff, so the risk of flooding is relatively high in barren areas. As is the case with surface properties, the amount of vegetation can change for many reasons, including those related to human land use (see next item).
Human activity and land use
It would not surprise anyone to hear that people modify what happens on Earth; supporting evidence is not difficult to see if you step outside. In the case of water storage and movement, two modern phenomena are particularly important. First, consider a river that is downhill from a suburban neighborhood. You might imagine that in the days before humans, the area was forested and dominated by permeable soils and rocks (Figure 7.16., left). After the impermeable roads and buildings appeared, runoff become far more important than it once was, and flooding of the river and downstream regions became more frequent (Figure 7.16., right).
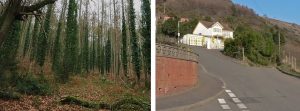
This common phenomenon has had a profound impact on human and natural communities alike. Second, the clearing of land for agriculture (Chapter 9), forestry (Chapter 12), and other purposes has also increased flood risk along many waterways. If plants in a field or forest are removed, water will move more rapidly along the surface than it once did. In short, although flooding is clearly a natural hazard, humans can increase its effect.
Prediction. As we saw for the other natural sources of stress on this list, we can assess the likelihood of flooding in both the near and far term. Weather forecasts of heavy rainfall can provide information about the immediate threat (i.e., days or weeks) of flooding, and appropriate preparations can be made in response. In the long run, analysis of the history of a region can be used to generate a flood zone map showing the probability that areas will flood. Typically, such a map is divided into 10-, 100-, and 1000-year flood zones, in essence, the zones with a 1 / 10, 1 / 100, and 1 / 1000 chance of flooding each year (Figure 7.17).
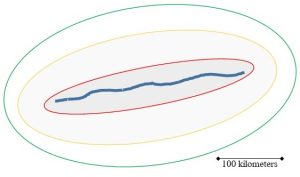
As these maps are based on statistics and probability, they are subject to some uncertainty. Furthermore, and very importantly, they predict events for each year. An occurrence of a large flood now does not guarantee anything about the future. So, for example, just because houses in the 100-year flood zone are under water in 2023 their owners should not assume they are free and clear until 2123! The chance that a flood of the same size will occur in 2024 is still 1 / 100.
Prevention. Clearly, we cannot change the weather and other natural processes contributing to flooding. However, we have the power to minimize both the occurrence of and damage from flooding in several ways.
Land use choices
The seemingly short and simple message here is: do not live in flood zones. The maps noted in the paragraph above are intended to inform development decisions, although people still build in risky areas (Box 7.2 explores “why??”). Generally, property owners in flood-prone regions are subject to laws requiring the purchase of additional (and costly) hazard insurance. Still, since public resources (i.e., taxpayer dollars) are often used to clean up after floods, there is considerable debate about how or even whether any kind of development should be allowed in such zones.
Box 7.2. Why build in a flood zone?
You might wonder what would motivate someone to build a home or other structure in an area that is likely to flood in the foreseeable future. Well, some of those locations are attractive enough for people to take the risk, although it is very often a move that leads to loss of property, money, and life. Coastal zones, for example, have obvious appeal for those who like to live or visit the ocean. As we saw in the section on erosion above, though, beaches and barrier islands, popular places to construct hotels and houses, are very unstable. They are also vulnerable to flooding. Floods of inland rivers can cause massive damage and destruction as well, yet people live in these risky areas nonetheless. To understand why, it is helpful to examine a diagram of a river and its immediate surroundings (Figure 7.18).
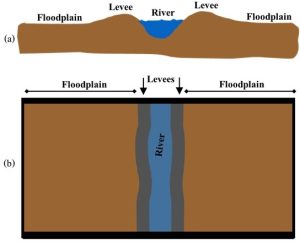
Under normal conditions, a river is confined to its channel. However, when more water enters the system than can be contained, there is overflow onto the adjacent land. This area on either side of a river is called a flood plain and is typically very flat and fertile. How did it get that way? The short answer is: repeated flooding. Each time the water exceeds the capacity of its channel it flows across the plain, depositing layers of sediments that were previously carried in the river. So, flooding creates a zone that is great for building houses because it is flat and for farming because its soil is full of nutrients (more about factors affecting farming is described in Chapter 9). Note that in addition to creating a flat plain, flooding also produces natural levees on either side of a river, structures that run parallel to the channel. These are made of coarse sediments that get piled up on the banks by flowing water. Each time there is a flood, levees—essentially walls of soil and rock—get larger and the height to which the water must rise to enter the flood plain increases. Humans can modify levees to make them even higher, although under the intense stress of a strong hurricane or other event they can fail, allowing a large amount of water to quickly enter the flood plain (this is a common cause of flood-related damage to humans and their structures).
Build structures to protect
Sometimes, communities try to reduce risks by constructing walls and similar structures. For example, an artificial levee or a dike can be built to keep water from damaging homes in flood-prone areas. Unfortunately, these walls tend to fail under extreme stress from very high and fast-moving water, in other words, when they are most needed. Additionally, their presence can encourage people to live in risky flood zones that might otherwise be considered uninhabitable. For example, New Orleans, Louisiana, U.S.A. suffered severe damage in 2005 when rising waters associated with the very powerful Hurricane Katrina broke through levees built to only withstand milder storms.
7.3. BIOLOGICAL STRESSORS
Stress from biological entities adds to the pressures exerted by non-living hazards. Chapter 5 describes the details of the three deleterious interactions among organisms listed below, so we will not dwell on them again here. You should keep in mind that, as we saw with the physical stressors above, living hazards are extremely important to members of today’s biosphere and also challenged and shaped life on Earth long before humans arrived.
7.3.1. Competition
Competitors are denied easy access to limited resources by the presence of each other. Ultimately, the weakest individuals die as a result of this phenomenon. Both participants are harmed, or endure stress, during competition (Chapter 6).
7.3.2. Predation
Here, a predator receives benefits from eating its prey. This type of stress affects both participants, though, because only the most fit individuals—that is, relative to both catching food and surviving predation—will endure long enough to produce offspring (Chapter 5).
7.3.3. Parasitism
Like predation, this interaction is characterized by a winner and a loser: a smaller parasite harms a larger host by causing disease or other adverse outcomes. Disease can kill directly by bringing on lethal changes or indirectly by weakening a host. In the latter case, since its fitness is reduced, the affected host is less likely to reproduce. See Chapter 5 for more details about this important stressor.
THE CHAPTER ESSENCE IN BRIEF [2]
The distinction between natural and anthropogenic stressors is important because humans have little or no control over the former but a great deal of control over the latter. Earth’s hazards may threaten modern civilization, but informed decision-making can minimize the potential damage caused by tectonic, water-based, and biological stressors.
Think about it some more…[3]
Why do we distinguish between anthropogenic and natural hazards?
Which of our natural hazards worries you the most? What variables might affect your answer?
How does human land-use planning influence the severity of the damage caused by the natural hazards in Chapter 7?
Additional suggested reading on physical stressors
The U.S. Geological Survey has an excellent web page you can explore for a lot more information about Earth’s hazards.
- Some of those gases are directly toxic; carbon dioxide, which also can be emitted, kills because it displaces dioxygen and leads to the asphyxiation of aerobic organisms. ↵
- As you will find throughout this book, here is very succinct summary of the major themes and conclusions of Chapter 7 distilled down to a few sentences and fit to be printed on a t-shirt or posted to social media. ↵
- These questions should not be viewed as an exhaustive review of this chapter; rather, they are intended to provide a starting point for you to contemplate and synthesize some important ideas you have learned so far. ↵
A surface along which there is displacement of rocks. The movement is generally sudden and results from tectonic stress. Movement along faults can cause earthquakes. See Chapter 7 for more.
Rain, snow, ice, etc., that has a pH lower than is natural. It can be caused by natural and human activity. See Chapter 10 for more.
In geology, a convergent plate boundary at which more dense crust slides below less dense crust. See Chapter 3 for more.
A type of mass wasting event in which material moves downhill; in this case, the falling rocks, etc., do not contact the Earth's surface as they descend. See Chapter 7 for more.
When all the trees in an area are cut down at one time. See Chapters 7 and 12 for more.
In geology, a term that refers to chemical and physical processes that break down and transform rocks and the remains of living materials. Weathering products can be transported and incorporated into new rocks and soils. Compare to erosion. See Chapter 3 for more.
Refers to processes that transport the products of erosion. Typically, sediments are carried in water, wind, or ice. Compare to weathering. See Chapter 3 for more.
In ecology, the recovery of an ecosystem after a relatively minor disturbance. Some remnants of previous communities are present. Compare to primary succession. See Chapter 5 for more.