1 An Introduction to Interdisciplinary Environmental Science
Jason Kelsey
Environmental science is a term familiar to most modern audiences, but its meaning often is misunderstood. Some people equate it with activism, protests, and rallies about preservation of wild areas and organisms. Others see it as a simple set of rules about how we should separate clear from green glass, turn off lights in vacant rooms, use less water, and buy recycled paper. Such assumptions lead many to conclude that environmental scientists are opponents to business, technology, freedom, and, frankly, good times. It turns out that little about these or related views is accurate, as we will soon see.
Key concepts
After reading Chapter 1, you should understand the following:
- The scope of environmental science
- The distinction between objective environmental science and subjective environmentalism
- How environmental science developed as a field
- The storage, movement, and finite nature of Earth’s materials
- How environmental science informs the principles and goals of sustainability
- The meaning and relevance of the term environment
- How organisms interact with their environments
- The importance and roles of the principle of environmental unity, the precautionary principle, and cost-benefit analysis
- The complicated relationship between Earth and humans
1.1. DEFINITION OF ENVIRONMENTAL SCIENCE
What, then, is environmental science? We begin with a seemingly straight-forward answer: it is the study of Earth’s natural systems and the forces affecting them. It is characterized by several important features.
1.1.1. It has a broad scope
If you stop and think about it, you will realize the enormous scope and complexity of this field, because by “Earth’s natural systems” we mean nothing short of all the living and non-living entities on this planet. Several sciences and their tools are clearly required if we are to take on this giant task, most notably biology, ecology, chemistry, geology, and physics. Put another way, it is very much a multidisciplinary endeavor. In addition, though, since the sciences on our list do not remain separate, rather they are combined in new ways to help address questions unique to our field, environmental science is interdisciplinary. By drawing upon and blending many disciplines, environmental scientists can study various issues, for example the ways organisms interact with their surroundings, how critical resources are produced, cycled, and transformed, and how human activity influences Earth and its many systems (including the quality of life for people).
1.1.2. It is objective
Environmental scientists strive to increase our knowledge and understanding of Earth’s natural systems. Put succinctly, we observe phenomena, collect evidence, and report findings and data—that is, we are objective in our approach. We must not interfere with or offer subjective, values-based judgements about what we think ought to be true. Note how our science is not the same as the similar-sounding environmentalism; the later term refers to a type of advocacy driven by a sense of right and wrong and a desire to protect nature from human activity (Figure 1.1 suggests one way environmental scientists and environmentalists differ).
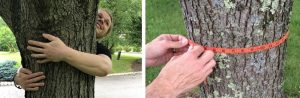
Objectivity is a particularly critical characteristic for us to consider because, unlike fields such as chemistry or physics, many people assume environmental science is a matter of opinion. It is rare to hear chemists accused of bias when they describe the workings of an atom or the amount of energy required to heat a given volume of water, say, but when an environmental scientist speaks about pollution, extinction, or climate, they will often be subject to criticism for what are perceived to be politically motivated conclusions. As we proceed through this book, you should learn to recognize the difference between approaches that are scientific and those that are non-scientific. We will see much more about science as a way of knowing and what distinguishes it in Chapter 2.
1.1.3. It is a relatively new field
People have been paying attention to the world around them for a lot longer than this, but a field known formally as “environmental science” did not really come into being until the 1960s. Biology, chemistry, and the rest mentioned above have arguably been around for centuries, so environmental science represents a novel way to study the Earth. Just what sparked the formation of this new field? The answer is complicated, but it is tied to the birth of what is known as the modern environmental movement. Many events, including the industrial revolution, development of assembly lines, urbanization, and large-scale agriculture, made people increasingly conscious of the potential adverse effects humans can have on the Earth and its systems. The widespread use of synthetic pesticides (Chapter 9) that began in the 1940s, though, had a particularly profound effect. Put very briefly, some people began to worry that these chemicals designed to kill unwanted insects could also affect organisms we value. Many people played roles in this increased awareness, but one of the most important was a woman named Rachel Carson. Her 1962 book, Silent Spring, was a fictionalized tale of a future with no more songbirds (hence the book’s title). What was her villain? Indiscriminate use of pesticides such as DDT, which through various mechanisms we will explore in Chapter 15, killed birds. The reaction to Carson’s work was strong, with many in industry (particularly those who manufactured and sold pesticides) quite critical of both her science and politics. She was publicly vilified and ridiculed by some powerful people, although others embraced her message and began to study the processes about which she warned. She died shortly after the book was published, but she was ultimately vindicated by the work of a presidential scientific commission. Additionally, the formation of the United States Environmental Protection Agency in 1970 and the U.S. ban on the use of DDT in 1972 were influenced by Carson’s book and her followers.
1.1.4. It is highly relevant and often newsworthy
Environmental scientists have become increasingly visible during the past several decades because they are generally called upon to study and fix environmental damage caused by human activity. Since the 1960s, they have worked on many dramatic and highly publicized incidents involving the exposure of people to poisons. It is by no means an exhaustive list, but some of the most noteworthy are briefly described here.
Cayahoga River, Cleveland, OH, U.S.A., 1969
This river was so polluted by industrial waste that its surface caught fire, capturing the attention of the public and highlighting some of the risks associated with unregulated dumping of hazardous substances into water.
Love Canal, City of Niagara Falls, NY, U.S.A., 1976 – 1977
This event was a defining moment in the environmental movement of the 1970s. Very briefly, hazardous waste was dumped into an unused canal between 1920 and 1953 and then buried. The area was developed into a neighborhood, including a public school, and seemingly no attention was given to the potential hidden risks beneath the surface. All was well until very heavy rains started to expose the buried materials in 1976. The public was horrified by media images of school children fleeing from noxious odors, the melting of tires of cars parked in chemical puddles, and the idea that careless (or worse) management of industrial products could threaten human lives. The effects were exaggerated by some, including reports of multiple deaths that never occurred; nevertheless, a serious health crisis ensued. Shortly thereafter, the place was deemed uninhabitable, and it was purchased by the United States government. An expensive clean-up was undertaken, paid for by a combination of federal and industry funds, and nearly 30 years later most of the neighborhood was reopened (Figure 1.2 is a photo of a barrier and a warning sign posted during the site remediation). The aftermath of this event included protests, suspicion, lobbying, and the enactment of many new laws controlling hazardous waste management (see Table 1.1 for some examples). Environmental science as a field also became noticeably more important.
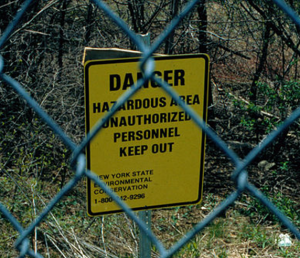
Table 1.1. A sampling of some important environmental regulations enacted in the United States since 1960.
Name of law | Summary | Year |
National Environmental Policy Act | Established guidelines for the construction of federal facilities to minimize adverse environmental consequences. | 1969 |
U.S. Environmental Protection Agency (EPA) founded | A federal agency was created to monitor and regulate the release of toxic substances into soil, air, and water. | 1970 |
Clean Water Act (amendments) | Established in 1948; amendments of 1972 brought sweeping changes. It created rules governing the amount and types of substances released into natural waters. | 1972 |
Endangered Species Act | Established criteria for defining organisms at risk for becoming extinct and guidelines for their protection. | 1973 |
Clean Air Act | Established rules governing substances released into the atmosphere. Subsequence amendments expanded it. | 1973 |
Resource Conservation and Recovery Act (RCRA) AND The Toxic Substances Control Act (TSCA) | Established definitions and guidelines on record keeping, storage, transportation, and disposal of hazardous and other wastes to prevent environmental contamination; outlined roles and responsibilities of waste generators and handlers. | 1976 |
Comprehensive Environmental Response, Compensation, and Liability Act (Superfund) | Established rules governing disposal and management of hazardous waste and created a fund to help clean up polluted sites. | 1980 |
Marine Protection, Research, and Sanctuaries Act | Restricted and regulated dumping of potentially hazardous substances into the world’s oceans. | 1988 |
Oil Pollution Act | Strengthened guidelines regarding oil spill clean up. | 1990 |
Energy Policy Act | Established rules governing the manufacturing and storage of fuels and other power sources; set aside funds for development of non-fossil fuel sources. | 2005 |
Three Mile Island nuclear power plant, near Harrisburg, PA, U.S.A., 1979
This extremely important event continues to exert influence over public policy and opinion in the United States. In short, an accident led to the release of some radioactive material into the surrounding area. Although it could have been much worse, the event was serious, sending shockwaves through the public, bolstering the anti-nuclear movement, and creating an enormous amount of suspicion about such facilities. In fact, no new nuclear power plants were brought on line in the U.S. after the accident (until 2023, as we will see in Chapter 10). Part of the Three Mile Island site was eventually brought back on line, and it continues to generate electricity today. Two other accidents in subsequent years—at Chernobyl, former USSR in 1986, and Fukushima, Japan in 2011—were far more destructive, leading to substantial releases of radioactive materials into Earth’s atmosphere and waters. The accident in 1986 led directly to about 30 human fatalities, and thousands more are thought to be at risk of death from chronic diseases like cancer. The regions surrounding both sites continue to be sufficiently contaminated to pose health risks (even the 30-year-old one at Chernobyl). In addition, the 2011 accident has been linked to elevated levels of radiation in marine life. Worldwide response to these incidents was intense: some countries using it declared they will phase nuclear power out of their energy grid in the coming years, and others who do not currently use it say they will remain nuclear free indefinitely.
Bhopal, Madhya Pradesh, India, 1984
A chemical plant owned by Union Carbide released large amounts of methyl isocyanate gas (and probably others) that sickened over 500,000 people. On the order of 20,000 deaths have been linked to the event. The cause of the release is subject to some debate, with locals citing poor, even criminal, plant management, whereas corporate officials claim it was caused by sabotage. In any case, much legal action followed in the decades afterward; Union Carbide paid out a large sum of money and a handful of company employees were convicted of crimes. What the long-term health effects will be remains unclear, and environmental scientists continue to study it. Suspicion and animosity persist to this day.
Prince William Sound, Alaska, U.S.A., 1989
The oil tanker Exxon Valdez ran aground and released nearly 11 million gallons of oil into a pristine ocean ecosystem. Images of oiled beaches and animals led to intense public outcry, questions about the wisdom of oil exploration in environmentally sensitive areas, and increased scrutiny of the petroleum industry (Figure 1.3). Several changes in U.S. government policy regarding responses to oil spills were enacted as a result. In addition, environmental scientists took the opportunity to study the effects of oil on organisms and ecosystems as well as new strategies to clean up after such an accident.
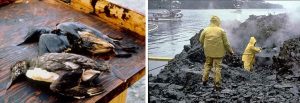
In 2010, an explosion on an oil platform in the Gulf of Mexico caused human deaths and an even larger release of oil into the ocean than that seen in 1989, raising additional questions about the responsibility industry has to protect natural environments.
Bozinta Mare, Romania, 2000
A release of cyanide led to the deaths of hundreds of tons of fish in the Somes River; the toxin eventually made its way into the Tisza and Danube Rivers. The fishing industry was adversely affected as a result, and the usual questions and suspicions about industry were raised. Environmental scientists often study these types of events because they affect both natural and human economic systems.
Northern portions of Gulf of Mexico, U.S.A., ongoing
Releases of agricultural waste into the Mississippi River contribute to the formation of a so-called dead zone in the Gulf. The presence of certain fertilizers can cause rapid depletion of oxygen in water and the deaths of high numbers of fish (Figure 1.4 shows the results of a typical fish kill). This phenomenon has captured some attention during the past several years, particularly among people who make their living off commercial fishing. Environmental scientists devote a substantial amount of energy to studying this problem, and we will see more about it in Chapters 4 and 5.
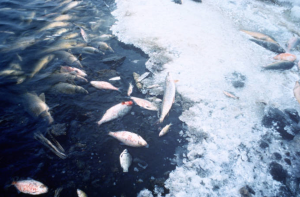
Declines in the population sizes of certain organisms, worldwide, ongoing
The previous examples on this list demonstrate how pollution can affect human health and economic wellbeing. A different kind of issue also is within the realm of environmental science, though: factors affecting the extinction of organisms that are not human. As we will see in detail in Chapter 6, many forces, both natural and of human design, can threaten the continued success and existence of organisms on Earth. Often, environmental scientists are called upon to study the likelihood that a particular species will go extinct and to make recommendations about how to protect it if it is judged to be endangered.
The potential that DDT has to bring about extinction of certain birds (described above) is one example of the way scientific data were used to protect organisms. In fact, bald eagles and other large predatory birds nearly became extinct in the 1970s, but the steps recommended by environmental scientists helped save them. Many other specific examples of such work could be cited, but they are beyond the scope of this textbook. What is important for us, though, is a common theme that connects most cases involving endangered species, namely, the conflict between protection and human activities (e.g., recreation, farming, manufacturing, development). Quite often a group or groups of people oppose putting an organism on the endangered species list because that action would require them to change their lifestyles in subtle or even dramatic ways. Questions about the relative importance of non-human organisms can lead to heated debates both in- and outside of legislatures. In these instances, environmental scientists may find themselves in an uncomfortable position as they present evidence of the likely adverse effects that a particular extinction could bring about to natural systems. People facing economic hardship or other losses in the name of protection of an animal or plant can be a rather difficult audience to persuade!
1.1.5. Its importance keeps growing
Environmental science is critical to public policy makers, academia, and industry. Among other consequences of the events described in the previous section, the United States government has passed many laws governing toxic and radioactive substances (refer back to Table 1.1). Public opinion certainly played an important role, though scientific data were indispensable to the development of legislation. Beginning in the 1970s, environmental science became an academic major that students could readily study in college, increasing the number of people working in the field. In the 1980s, a large industry grew to help affected companies interpret new and rapidly changing government regulations. The 1990s and 2000s saw additional needs and opportunities as problems related to waste management, energy resources, and air pollution became more and more urgent (we will learn about these and many other topics in upcoming chapters). It would be overly simplistic and inaccurate to suggest that the trend from the middle of 1900s to the current day can be characterized by ever-increasing (or even constant) public and governmental interest in environmental protection. To be sure, there have been ebbs and flows in the amount of energy devoted to these issues. However, it is fair to say that things are dramatically different now than they were sixty years ago, and environmental science has evolved and grown into a mature and highly relevant field.
1.2. FUNDAMENTAL PRINCIPLES IN ENVIRONMENTAL SCIENCE
The field of environmental science, as well as our approach to it, is built upon several principles. We will refer to these throughout this book, so you should become familiar with them early.
1.2.1. Earth is closed with respect to materials
This concept will recur more often than any other on our list. In short, it can be understood to mean that materials will neither enter nor leave Earth’s system (for all intents and purposes—trivial amounts of material do come and go occasionally). Note that Earth is open with respect to energy (Chapter 4).
Two important consequences
1. Material resources are finite. We cannot count on additional inputs of any resources from outside of Earth; instead, we must rely on either what is currently here or on processes that can convert spent materials back into usable forms again. Clearly, many items are important to us, including nutrients, water, soil, breathable air, building materials, fuels—to name just a few—and our continued survival depends on an understanding of the factors controlling their availability as well as our effective management of their supply. This book will focus a great deal of attention on the many natural and human-based ways resources are made accessible to organisms, utilized, replenished, and recycled.
2. Waste products must be managed. Contrary to the language we might use when dealing with our trash, it does not go “away” after we pitch it into a can. In fact, unwanted waste can persist long after we are done with it if it is buried under ground. Even incineration, which can change its form, still releases products that must be dealt with. As we will see in detail in Chapter 13, appropriate waste management is critical to environmental quality.
1.2.2. We keep track of materials
Whether or not demands for vital resources can be met is very important to environmental scientists, and knowledge of factors affecting the whereabouts of scarce materials helps answer two fundamental questions.
Where is the material and how does it move?
Reservoirs. As we will learn in upcoming chapters, the materials we value and need can be found in a number of places on this planet. Put into language used by environmental scientists, materials are stored in reservoirs. Note that here we refer only to naturally occurring reservoirs, not tanks, buildings, and others constructed by humans. We will return to this point shortly.
Pathways. Earth is by no means static—materials do not just remain in their reservoirs. Instead, they move around, sometimes undergoing dramatic changes while doing so, via processes referred to as pathways (more in upcoming chapters). Figure 1.5 is an idealized way to visualize the reservoirs and pathways for any material.
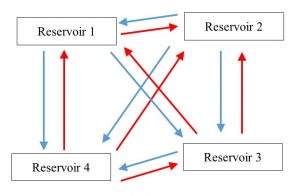
Is there enough to go around? We often want to know about reservoirs and pathways because they affect availability of materials we desire. Imagine that you are designing a new housing development and want to know if the amount of water in a local reservoir will meet the demand of all the people who would live nearby. For the sake of argument, say your project is to be constructed near Reservoir 1 in Figure 1.5, above. You could measure how much water is currently stored there, but you need to account for declining availability that would occur after you start to tap into it (Chapters 4 and 11). Whether water demand can be met into the future depends on how quickly it moves along pathways from the other three reservoirs back to Reservoir 1. The wisdom and feasibility of making this investment in new housing would be heavily influenced by the data provided by environmental scientists working at the site.
1.2.3. There are limits on how many organisms Earth can support
Since Earth’s physical resources, including space, food, and water, are limited, only a finite number of living things can occupy this planet at any one moment in time. Now, although some of the factors influencing that number are objectively measurable, there is still some disagreement about the upper limit for life on this planet, particularly how many humans can plausibly live here (more below and in Chapter 8). Nevertheless, scientists generally agree that we would experience adverse consequences if human numbers and activities exceeded the Earth’s ability to support us. Here we introduce two concepts related to resource availability and what is needed to maintain populations of organisms.
Sustainability
This term is often used to describe phenomena in the realm of environmental science and also has taken on a certain amount of popularity among the general public. It is broadly related to whether a process or activity can continue indefinitely, or endure unchanged, into the future. The concept is often framed in human terms and posed as a question such as: how much of a given material can we use today without changing the access of subsequent human generations to that same material? The exploitation of vital resources such as water, fertile soil, fuels, animals and plants, and land can all be subjected to this assessment. Upcoming chapters will focus on how these and other issues can be viewed through the lens of sustainability.
Carrying capacity
Here we see an application of sustainability that is widely used by environmental scientists. It is defined as the maximum number of individuals of a species (more on the species concept will be presented in Chapter 5) that can live in an environment without reducing the ability of that environment to support the same number—with the same quality of life—in the future. Two examples will help illustrate this idea. In the first, we want to know the carrying capacity of white-tailed deer in a certain forest. We could quantify the amount of food, water, and space each individual needs, as well as how much of each of those requirements are met by the environment, and come up with a number. We might do our research, make our calculations, and conclude the carrying capacity in this case is 75 animals. If the size of the current population is higher than 75, say 100 for the sake of argument, the environment will be degraded, food will be eaten faster than it can regrow, and future deer will not have access to the same amount and quality of resources as today’s (Figure 1.6).
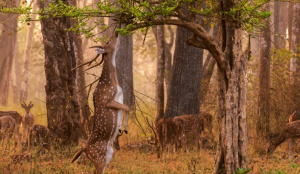
Numbers will therefore dwindle in subsequent generations. In the second we ask what is the carrying capacity of Earth for human beings? It turns out this question is far more difficult to answer than was the one about deer because there are so many unknowns. Although we could certainly determine how much food, air, water, and so forth are required to keep someone alive, we can only speculate about how much a typical person will use above and beyond their basic needs. Differences in standards of living across Earth, and just how many resources people will demand in the future, confound our ability to reliably calculate human carrying capacity. In fact, a rather wide range of answers have been offered by various researchers. Some conclude that the upper limit is about 2 billion people if everybody in the world lived a lifestyle akin to that of an average middle class person in a country like the United States, whereas a maximum of 40 billion could be sustained if every person used only enough resources necessary for basic survival (note the current population is about 8 billion people, and according to United Nations projections, is likely to grow to nearly 10 billion by about 2050[1]). Presumably, the answer lies somewhere between those extremes (close to 10 billion is a typical estimate offered these days), but, given the uniqueness of the human race, it is a difficult number to derive. We will explore much more about factors affecting the growth of Earth’s human population in Chapter 8.
1.2.4. Organisms are linked to their environments
The title of this book contains the word “Environment” for a reason: it is a central concept, one critical to our understanding of living things. Here we begin to explore how it is important to our studies.
What is an environment?
This word is often misused and misunderstood. Contrary to popular belief, it does not mean all the forests, lakes, oceans, and rest of Earth’s outdoor places, nor should it be used to refer to natural resources or other non-human spaces. Its formal definition is composed of two major points.
1. An environment is a space. Simply put, it can be thought of as the location in which an organism lives, or at least temporarily resides.
2. An environment is made up of those things that affect an organism. In addition to providing the space in which it exists, it influences and shapes an individual. It can be visualized as the set of forces that applies pressure, or stress, to living things.
So, now we know that when someone says “I care about The Environment” they should instead say something like “I care about Earth’s natural environments”. The important point is: there is no single environment. Instead, many, many environments can be identified. We will see some specific examples of entities that can be categorized as environments shortly.
What environmental factors influence organisms?
Some of the specific environmental factors of importance to organisms are temperature, presence of sunlight, moisture availability, types and amounts of food sources, altitude, chemical properties, and the presence and activities of other organisms. These and other variables control which organisms are present in a particular place. One of the underlying principles for us is the notion that organisms do what they do in response to their environments—put another way, they adapt to the conditions in their habitat. One obvious example would be the strategies fish have adapted to live in water: gills, fins, and so forth allow them to succeed in their environment. Oak trees provide another helpful example. They are adapted to environments with abundant rainfall and rich soil, whereas cacti can tolerate and even thrive in dry deserts, places which would be deadly to the oaks. Lions, seals, snakes, sharks and the rest of the organisms on Earth all have successfully responded to the pressures applied to them—their continued existence here suggests as much. Furthermore, since environments are not constant, the most successful organisms are the ones that can change as necessary. Those that cannot alter their life strategies will fail, likely becoming extinct (more in Chapter 6). A final note is worth considering here: environments play critical roles in shaping the development and defining features of human beings as much as they do other organisms. In our case, environment is quite complex, containing the basic features related to survival listed above, but also includes social forces like family, friends, economic conditions, education, and the like. Who you become, how you appear, and what you ultimately do, are all influenced by those forces acting on you during your life. Of course, innate features affect organisms as well, as we will see in Chapters 5 and 6.
Can organisms affect their environments?
The short answer to the question is: yes. Just by its presence, an organism will change the place in which it lives. Animals eat plants, sometimes take down entire trees, and burrow in soil, and plants remove nutrients and water from soil in which they are rooted. Organisms even affect the composition of Earth’s atmosphere, adding biologically usable oxygen gas to it (see Box 1.1 for more about this crucial process and its consequences). And the list goes on, containing both familiar and unfamiliar ways environments are shaped by living things. We will see many examples of this phenomenon as we make our way through this book.
One of the most fundamental environmental changes brought about by organisms is related to the Earth’s atmosphere. As you likely realize, many organisms, including we humans, require dioxygen gas (O2) for basic survival. The fact that breathable oxygen has not always been available on Earth might surprise you, though. How do scientists know anything about conditions of the past? Through many studies of evidence stored in rocks, soils, and fossils, they have concluded that the composition of the early atmosphere was different than that of today. Notably, that crucial O2 gas was not present. Yes, there was oxygen here, but it was stored in reservoirs other than the atmosphere. Now, some organisms, known as anaerobes, do not need oxygen to survive—in fact, they are poisoned by it—so they dominated the planet in the early days. Put another way, they were well adapted to their no-oxygen environment. Over two billion years ago, certain ancient organisms that release oxygen gas as a product of their normal activities began to appear (more about these organisms is presented in Chapter 5). It took a very long time, as we will see in Chapters 3 and 6, but eventually the atmosphere was altered such that it contained appreciable amounts of oxygen. Then what happened? Well, organisms adapted to life without oxygen were in trouble. They had to change their strategy, find environments that were free of oxygen (such places still exist today), or go extinct. Those that could use oxygen, aerobes, gained a big advantage and began to develop from small, relatively simple individual cells, into large, complex, organisms such as animals and plants. The tremendous diversity of living things we see today (Chapter 6) is very much a product of widely available oxygen. Again, organisms affected their environments, and those changes in turn affected other organisms. The relationship between the non-living components of Earth and life is complicated, dynamic, and one that brings about changes on both sides.
Where can environments be found?
They are everywhere. Any entity that meets the criteria we listed above, that is, it surrounds and influences organisms, can be defined as an environment. Size, shape, complexity, or whether it is natural or the result of human actions are irrelevant considerations. In other words, the possibilities are seemingly limitless. Since the space available to us in these pages is limited, though, we will consider only a small number of examples
A forest. To many people, this or a similar place comes to mind when they hear or use the term “environment”, for it contains all the components commonly associated with the (incorrect!) usage of the term: trees, animals, streams, and natural processes (Figure 1.7). To us, though, it is a type of environment with characteristic features and conditions appropriate to support the organisms living there. A list of the specific and influential environmental properties found in a forest in, say, northern New York State (U.S.A.), would include a land-based area (vs. under water), abundant rainfall, temperatures that range from near 38 °C (about 100 °F) in the summer to ˗20 °C (about ˗5 °F) or lower in the winter, and sunlight that varies seasonally. The living things present there will be those adapted to the prevailing conditions. Now, if one or more environmental properties change, the organisms that dominate will also change (Chapters 5 and 6).
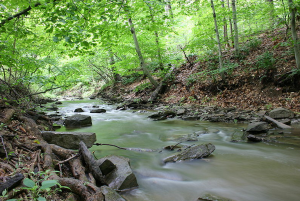
A desert. Although also land based, this environment is very different from a forest. Importantly, a desert receives far less precipitation (Chapter 5). Wide temperature swings between very hot days and cool nights are also likely. Instead of trees, dominant organisms would include cacti, sagebrush, and animals that are able to survive there (Figure 1.8).
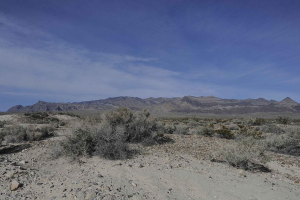
A lake. Clearly, this water-based environment is fundamentally different from the first two on our list (Figure 1.9). Here organisms will be adapted to obtaining food and oxygen from relatively still, fresh water (not moving, as in a river, or salty, as in an ocean). If this is a northern lake that freezes in the winter, it will be dominated by different organisms than one in, say, Sub-Saharan Africa, a place that does not freeze.
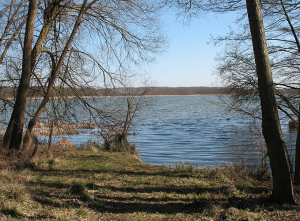
A classroom. Although not naturally occurring, this space meets our criteria for inclusion here: organisms can live in it (some non-human ones even live there full time) and are influenced by its conditions (Figure 1.10). Properties related to temperature, humidity, odors, light, and food availability could all be described. A room in the basement of an academic building might be damp and warm, encouraging the growth of mold on its ceilings (as evidenced by brown or black splotches). A room on the fifth floor of the same building could be dry and hot, discouraging mold but supporting flies and other insects. Both areas would be teeming with unseen bacteria (see Chapter 3 for more about these ubiquitous and important organisms), although the different conditions indicated would likely mean they would not be home to the same bacteria. What about the humans who come and go? Well, at a minimum the conditions are (we hope) appropriate enough for them to survive there for a few hours a week. Proper design would create an environment most conducive to learning. Chalkboards, technology, an instructor, and students also influence humans in the room. All these variables could contribute to different learning outcomes for different people. Put another way, environmental factors play an important role in shaping all organisms, even those spending time in artificially constructed spaces.
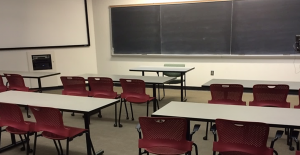
A person. The final example on our list might surprise you: an individual human being is an environment for large numbers of microscopic organisms (i.e., they are too small to be seen without the aid of a magnifier). Even a completely healthy person houses—in fact, depends on—trillions and trillions of bacteria and other microorganisms (see Chapter 3) on a regular and on-going basis.
Services performed by microorganisms for us
Digestion of certain foods. The human digestive tract is the home for microorganisms that can help us obtain nutrients from some of the plant material we ingest. In fact, without the help of these tiny partners, we would not be able to digest this food (more about many types of relationships among organisms can be found in Chapter 5).
Protection against some illnesses. Our bodies are attractive places for certain invading microbes, termed pathogens, that make us sick (see Chapter 3). They can find their way inside of us by multiple means, but most of the time enter through one of our permanent openings to the outside (e.g., mouth, nose, urinary / reproductive systems, eyes, ears) or through temporary wounds. Luckily for us, we have developed relationships with other microbes that occupy the tissues near some of those openings. Our mouths, for example, are absolutely teeming with these helpful microorganisms. Their presence makes it very difficult (but not impossible) for disease-causing organisms to get very far into our bodies; the locals are so well adapted to the conditions in their environment, as well as sufficiently abundant, that invaders often are simply outcompeted and fail to make us sick.
Factors affecting resident microorganisms
The specific identity of the occupants inside us depends in large part on, you guessed it, the environmental properties of our bodies. Those with the appropriate adaptations can thrive under the temperature and chemical conditions of a human. Unsurprisingly, then, changes would likely affect organisms living in our guts and other places. Keeping these ideas in mind, consider how we respond to a disease such as strep throat. When a certain species of bacteria overcomes our defenses it can cause a reasonably unpleasant ailment. It usually produces a tender, swollen, red throat and a fever. Why do we experience these symptoms? First, bacteria from the outside outcompete our normal resident bacteria, take up space, absorb nutrients, and generally irritate their adopted home. Second, fever is one way your body manipulates environmental conditions to inhibit the growth of harmful and unwelcome invaders. A pathogen adapted to survive at human body temperature (37 °C) will struggle and even die if subjected to a habitat maintained at 38 or 39 °C. Additionally, people sometimes ingest chemical substances known as antibiotics to kill bacteria. In other words, they make a change to the chemical conditions of their bodies to thwart unwanted organisms. Unfortunately, antibiotics can also harm many helpful bacteria, making it difficult to digest food and otherwise maintain good health. As long as those changes are temporary, though, most people are willing to accept the negative consequences (diarrhea is possible, for instance) in the interest of a cure. We will see more about the highly nuanced and fantastically important story of bacteria and antibiotics in Box 5.6, Chapter 5.
Everything on Earth is connected
Central to environmental science is the idea that, although we tend to break it up into smaller components for the purposes of study, Earth is one large and complex environment made up of interconnected and interdependent units. We can certainly define and describe the organisms or air or water on the planet, but we understand them to be parts of a greater whole. Furthermore, since everything is connected to everything else, a change in one component—no matter how small—will bring about changes to other, seemingly distant, components within Earth’s systems. You should realize that predicting the far-reaching effects of a relatively small-scale action can be quite difficult, and we often are stuck retracing our steps only after an unexpected, and very likely undesirable, change has been initiated. We will see many examples of this idea, the principle of environmental unity, in upcoming chapters.
Decision making is informed by caution and risk assessment
Consequential decisions about our environments and health, such as whether to proceed with the construction of a new highway, approve a new vaccine, use a new pesticide, or allow a new preservative in food, generally are based on two fundamental premises. First, new chemicals, technology, and the like are assumed to pose a risk to natural systems (as well as humans) until shown to be safe. Although this starting point, known as the precautionary principle, is not embraced by all environmental scientists, public opinion and regulations tend to be driven by it. Importantly, it lays the burden of proof on those who advocate (and are likely to profit from) the use of those new potential hazards. There is some debate over the appropriateness of this principle, as certain people view it as a waste of money and effort. Advocates of it, on the other hand, point out how its conservative approach generally protects public health. Second, some risks are acceptable as long as they are outweighed by the good an action brings about. A cost-benefit analysis is critical to the evaluation of the advisability of any initiative or change (see Box 1.2 for a timely application of this tool).
Box 1.2. The COVID-19 vaccine: fun with cost-benefit analysis!
The COVID-19 pandemic of 2020 (and beyond) likely needs no introduction. It brought about much suffering, disruption, and death, but it also led scientists to perform what was believed by many to be impossible: they developed multiple safe and effective vaccines in a matter of months, instead of the many years that is typical for such work. Those who were vaccinated have largely been able to resume normal activities with scant risk of contracting a serious case of the disease (i.e., after vaccination, a small number of people came down with mild illness, but life-threatening cases requiring hospitalization fell to near zero). Those who did not get the vaccine continued to be at substantial risk of a debilitating or lethal case of COVID. In fact, after vaccination began, unvaccinated individuals accounted for an increasing percentage of the life-threatening cases treated in hospitals. So, what motivated this second group? Why expose yourself to avoidable danger? The answer is complicated. Of course, some could not tolerate the vaccine for medical reasons, but many eligible people chose to skip the vaccine because they believed, despite a lack of supporting evidence, it would lead to some kind of dire consequences (including, but not limited to, loss of fertility, loss of personal freedom to unscrupulous scientists and government officials, and unforeseen health effects of a novel vaccine). Put another way, they felt its risks outweighed its benefits. Let’s interrogate their assumption about risks with data and do a quick cost-benefit analysis
To simplify things, we will look only at the situation in the United States. According to governmental data, there were 33.5 million cases and 600,000 COVID-19 deaths as of June, 2021 (an average of a bit under 2% of those infected died from the disease, although factors such as age and pre-existing conditions raised that number to 10% or more in some groups)[2]. To some, that rate seems trivial (families who lost loved ones likely view it differently). Serious long-term yet non-lethal effects of COVID are also possible (so-called ‘long COVID), however, including organ damage, blood clots, chronic fatigue, and ongoing respiratory distress (not to mention quirkier symptoms like loss of sense of taste and smell that can persist for months). Furthermore, by some estimates, risk of death in the months following infection rise precipitously. The benefit of receiving the vaccine can be summed up, then, as substantially reduced risk of death and other adverse consequences.
What are the dangers of the vaccination? If we consult data provided by the U.S. Centers for Disease Control and Prevention (U.S. CDC)[3], we see that the risks of it are quite low. Somewhere between 0.0002% and 0.0005% of people who got one of the vaccines suffered some kind of anaphylaxis shortly after getting their shot. About 0.0003% of those receiving the one-shot Johnson and Johnson vaccine were affected by abnormal blood clotting (36 individuals out of 11.7 million vaccinated). Overall, 0.0017% of those receiving one of the vaccines died afterward (somewhat more than 4000 out of 117 million). This statistic about mortality is not quite what it seems, though, because rules governing medical facilities require that deaths from any cause after vaccination must be reported. So, car accidents, drug overdoses, gunshots, drowning, cancer, and other agents are included in that number. In other words, there is no reason to believe that the vaccine was responsible for all or even the bulk of the deaths. Famously, many people did experience short-term discomfort following their shot, a problem that is more troubling to some than others (in any case, the symptoms were not linked to real degradation in health status).
To summarize (doing a little math): being very conservative and cautious, the risk of an unvaccinated person dying after contracting COVID-19 is at least 1000 times higher than the risk of dying after receiving the vaccine. You decide which course of action is likely to cost less.
1.2.5. Humans and Earth operate on different time scales
As we will see in detail in Chapter 3, scientific evidence suggests that Earth is on the order of 4.6 billion years old. Primitive human-like beings, on the other hand, arrived on the planet relatively recently and have been around less than 3 million years. Modern humans have only been present for some 200,000 years. Depending on how you want to define it, evidence of the first recognizable civilization dates back in the thousands of years, with the modern era beginning a century or so ago. Of course, an individual human generally lives less than a hundred years, and much of the business of living is measured in days, hours, and minutes. Given the constraints on our perspective, then, it can be difficult to comprehend the vast amounts of time necessary for many natural forces to shape Earth’s environments. In upcoming chapters we will study, among other topics, the formation of continents, oceans, rocks, and oil as well as the development and evolution of living things, even though they generally happen so slowly we cannot observe them in real time. Human existence is just too limited to directly experience much of what occurs on Earth.
The story gets more complicated, though. Despite the short history of our species and the very brief time each of us is alive, humans have become a powerful agent of change on Earth. The pace at which we modify our environments, use and move materials, synthesize novel substances, and generate waste products can be extremely rapid, dramatically outpacing analogous natural processes that have been active throughout the planet’s long history. Environmental scientists try to assess the potential short- and long-term consequences associated with human actions in the context of the disproportionately large ability we have to quickly bring about changes.
THE CHAPTER ESSENCE IN BRIEF [4]
Environmental scientists study Earth’s systems and the forces affecting them. It is objective, relevant, and founded on essential principles about the interconnectedness of living and non-living phenomena. Chapter 1 lays the important foundation for the rest of this textbook.
1.3. BEFORE WE PROCEED
We end Chapter 1 with a preview of things to come as well as some suggestions about how to maximize the usefulness of this textbook.
1.3.1. What to expect next
This book is divided into three parts. The first consists of only this and the next chapter and is included to provide an overview of the scope of environmental science (you just read that part) and some tools we will use throughout our study (Chapter 2). The second part (Chapters 3 ‒ 6) describes the basic processes that are active on Earth, with little attention given to any sources of stress (human or otherwise) affecting those systems. The third and final part examines many forces that can disrupt the basic processes described in Part Two. The majority of these later chapters focus on human activities, although some coverage of natural sources of stress are given in Chapter 7.
1.3.2. What you can do get the most out of your reading
This textbook is designed to present environmental science as a coherent story, and you should approach it with that in mind. It is certainly not a work of fiction, but you should still try to follow it more like it were a narrative than as a collection of disjointed facts. To be sure, you will encounter a lot of terms, definitions, and descriptions of concepts new to you. These are presented to enable you to learn the language of environmental science so you can both understand the story as well as get to a point where you can tell the story yourself. You are encouraged to look for connections among the topics within and among chapters; use the cross references provided to help you do this. For example, when you are reading Chapter 5 you will see extensive connections back to Chapter 2. If you look at them together you will both remind yourself of important concepts as well as broaden your understanding of the subject matter. Environmental science is fairly hierarchical, that is, early chapters present foundational concepts upon which later chapters depend. You could jump around and read things in a different order, but if you keep the intended organization in mind while you do so, you will enhance your ability to make sense of things.
Think about it some more…[5]
Are environmental advocacy groups best categorized as environmental scientists or environmentalists? Is it possible they could be both? Neither?
Why do environmental scientists keep such careful track of the reservoirs and pathways of important materials? What fundamental principle informs this work?
What is the nature of the relationship between organisms and their environments? Could that relationship change with time?
Could the deaths of thousands of sea birds in Alaska be connected to the construction of a new housing development near, say, Atlanta, GA? Think about the principle of environmental unity as you ponder your answer.
- United Nations Department of Economic and Social Affairs, Population Division. 2022. World Population Prospects 2022: Summary of Results. UN DESA/POP/2022/TR/No.3 ↵
- covid.cdc.gov/covid-data-tracker ↵
- cdc.gov/coronavirus/2019-ncov/vaccines/safety/adverse-events.html ↵
- As you will find throughout this book, here is very succinct summary of the major themes and conclusions of Chapter 1 distilled down to a few sentences and fit to be printed on a t-shirt or posted to social media. ↵
- These questions should not be viewed as an exhaustive review of this chapter; rather, they are intended to provide a starting point for you to contemplate and synthesize some important ideas you have learned so far. ↵
An approach to study used by scientists. It is characterized by observation of phenomena without imposing judgements or other biases on the results. Contrast with subjective. See Chapter 1 for more.
An approach that is characterized by values and biases; contrast with objective. See Chapter 1 for more.
Advocacy-based, subjective approach to protecting earth's natural systems; may or may not be informed by objective data or science. Compare to environmental science. See Chapter 1 for more.
Chemicals designed to kill or inhibit unwanted organisms; widely used in agriculture. See Chapters 1 and 9 for more.
Dichlorodiphenyltrichloroethane, 1,1′- (2,2,2-trichloroethylidene) bis(4-chlorobenzene), or DDT is a pesticide designed to kill insects (i.e., it is an insecticide). It was used widely in the 1940s, 1950s, and 1960s but banned in the U.S. and largely phased out of use in the early 1970s. See Chapters 1 and 15 for more.
U.S. federal agency tasked with protection of soil, air, water, and ecosystems. See Chapter 1 for more.
City in western New York State (USA) that became famous when hazardous waste buried beneath it came to the surface. National attention and outrage led to new environmental protection laws. See Chapter 1, especially Table 1.1, for more.
The site of a major nuclear accident in 1986. Located in Ukraine, this power plant exploded due to multiple user and design errors. The environmental effects of this accident persist to this day. See Chapter 1 for more.
Refers to a low-oxygen region of a body of water such as the Gulf of Mexico. Runoff from human activities such as agriculture introduces excess nutrients into the water; fish and other oxygen-requiring organisms die off in such a zone. See Chapter 1 for more.
A term that refers to all of the factors that surround, influence, and sustain an organism. Earth is made up of many different environments. See Chapter 1, for more.
In response to environmental pressures, organisms develop strategies that allow them to survive and reproduce in a particular area. 'Adaptations' refer to those characteristics that enable success. See Chapter 1, for more.
Two oxygen atoms are bonded to form O2, dioxygen. This is the gas required by aerobic organisms; it makes up approximately 20% of the gasses in the atmosphere. See Chapter 1, especially Box 1.1, and Chapters 3 and 4 for more.
More formally, 'aerobic organisms', they require dioxygen gas for survival. See Chapter 1, especially Box 1.1, for more.
In ecology, refers to a trait that helps an organism survive and reproduce better than competitors. See Chapters 1, 5, and 6 for more.
Refers to the many different kinds of microscopic organisms that are single celled and prokaryotic (i.e., do not possess distinct cellular organelles). Collectively, bacteria are extremely diverse, are the oldest organisms on Earth, and are critical to many environmental cycles and processes. See Chapter 3 for more.
Refers to organisms that are too small to be seen without the aid of some kind of magnifying lens (e.g., a microscope). Includes bacteria, protozoa, certain forms of algae and fungi, and viruses. See Chapters 1 and 5 for more.
Generally, a kind of chemical substance that kills living cells. In practice, 'antibiotic' refers to a drug designed to kill harmful bacteria without appreciably harming the host taking the drug. See Chapters 1 and 5 for more.