13 Waste Management
Jason Kelsey
Earth is closed with respect to materials, as we know very well by now. Thus far we have explored the first of the two consequences of this fundamental principle described in Chapter 1: the supply of living space, arable land, food, energy sources, forests, clean water, and other valued resources is not infinite. In Chapter 13 we will look at the second consequence, namely, that waste cannot be eliminated, rather, unwanted products generated by the billions of people on this planet must be managed within the confines of our system. In other words, when you throw something away it does not disappear—what you are really doing is sending your waste on a long journey that moves it among multiple receptacles, processing centers, and storage facilities.
Key concepts
After reading Chapter 13, you should understand the following:
- The many sources and types of human-generated waste
- How and why waste management was different in the past than it is today
- The costs, benefits, and challenges of the multiple modern strategies used to manage waste
- The meaning of cradle-to-grave management of hazardous waste
- Some of the strategies used to reduce waste generated by humans
- Why proper waste management is important
13.1 SOURCES OF WASTE
13.1.1. Natural
All living systems release waste as part of their normal functioning. Organisms extract nutrients from the food they ingest and then release unwanted products into their environments. Although some of those leftover materials are toxic to the individual releasing them, they will make a fine meal for another organism that is adapted to live off biological waste. Fungi and certain bacteria, for example, utilize remains and excrement to gain energy and materials they need. Of course, these decomposers also perform a critical function in that they free up carbon, nitrogen, phosphorus and the other elements vital to the continued survival of the biosphere (Chapter 4). Importantly, natural products rarely accumulate for extended periods but instead are fully recycled (with a few exceptions—we saw in Chapter 10 that petroleum, for example, persists in the lithosphere for hundreds of millions of years).
13.1.2. Anthropogenic
Human activities generate a great variety of waste. Some of these materials may be decomposed into simple products which return to Earth’s biogeochemical cycles (Chapter 4), but many are not so easily broken down by natural chemical reactions. As we will see shortly, the accumulation of anthropogenic substances can lead to several undesirable consequences, including exposure of human and natural systems to toxins, degradation of soil, air, and water, the reduction in available land appropriate for housing, farming, and other uses, and the removal of valuable resources from natural systems indefinitely. Because they pose different levels of risk and require different management approaches, anthropogenic waste is assigned to one of several categories.
Municipal solid waste (MSW)
Generally known as trash or garbage, this first group includes unwanted products people generate during their regular, daily routines. Relatively innocuous materials like glass, paper, plastic, fabric, and food scraps are among the constituents of MSW (consult Figure 13.1 to learn more). We will focus the bulk of our attention on this category of anthropogenic waste.
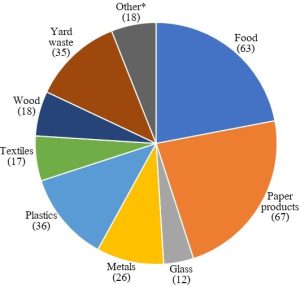
Hazardous waste
Solvents, metals, explosives, drugs, pesticides, radioactive materials, and many other potentially harmful substances are released from homes, offices, factories, hospitals, universities, and military facilities. These substances require special handling and cannot be combined with MSW.
Biomedical waste
Bodily fluids, tissues, infectious agents, and objects that have come into contact with these biological substances (syringes, broken glass, sample vials, bandages, and related items) are included here. Like hazardous waste, biomedical waste is subject to stricter rules than those for household trash.
13.2. PAST PRACTICES
The problems associated with human-generated waste were not always quite as pressing or complex as they are currently. Prior to the middle part of the 19th Century, the volume of waste generated was small and easy to handle relative to what we see today. The reasons for this difference are straight forward: far fewer people were present on Earth (between 1 and 2 billion, roughly 1/5th of the current population) and resource use per person was also generally lower due to limited access to technology and lower standards of living (review Chapter 8 for more information about the history of human population growth). For example, in the United States, both the number of people and the amount of trash generated per person increased steadily from 1960 to about 2000, although the number has levelled off in recent years (Figure 13.2).
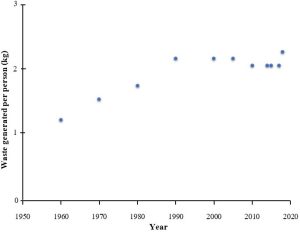
Furthermore, waste generated today is broadly more diverse (i.e., it contains many more objects with different properties) and more toxic than it was in the past, making modern management both increasingly difficult and urgent.
It would be fair to say that, until the middle parts of the 1900s, most people were guided by the familiar adages “dilution is the solution to pollution” and “out of sight, out of mind” (Chapter 11) when it came to managing their unwanted products: garbage, sewage, and so forth were routinely left in open pits, buried in shallow holes, pumped into surface and underground waters, burned, or moved to a remote or sparsely populated location. Four specific approaches are briefly described here. Note that, although replacements for them have been developed during the past several decades, these older methods persist. Abandoned sites can still exert adverse effects (more below) and, in some areas, they are still actively used.
13.2.1. Open dumps
Some people equate the term “dump” with “landfill,” although the two terms are far from synonymous. The former refers to a place in which many types of garbage are left uncovered on the Earth’s surface with few or no barriers, or containment structures, to restrict access to and movement of the trash away from the site (Figure 13.3).
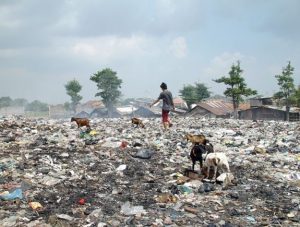
In other words, animals (including humans!) can scavenge in dumps, likely exposing themselves to hazardous substances, and toxic materials can readily flow via runoff and leaching to streams, lakes, oceans, and groundwater (Chapter 4 and Chapter 11). The later term, landfill, is associated with a modern approach to waste that has replaced dumps in many places, as we will see shortly.
13.2.2. Open burning
Another strategy used in the past was simply to ignite piles of household trash, tires, clothing, plastics, chemicals, and so forth. This practice, often referred to as open burning, is restricted in the United States by several laws linked to the Clean Air Act. You should realize, though, that this approach to waste management is still allowed (in the U.S. and elsewhere) under certain conditions.
13.2.3. Ocean dumping
Here, as the name suggests, trash is transported out to sea and released into the water (Figure 13.4). This strategy was once widespread, and many nations routinely discarded sewage, along with domestic, medical, construction, dredging, military, and even nuclear waste into the ocean.
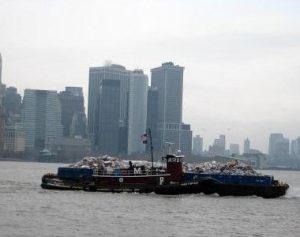
Laws enacted during the past several decades have reduced, if not eliminated, the release of trash into oceans. For example, the United States passed the Marine Protection, Research, and Sanctuaries Act in 1988 to regulate this practice. In short, it largely prohibits dumping of several kinds of waste (e.g., that from hospitals, industry, and sewage treatment facilities—Chapter 11), although exceptions are made for some materials and circumstances (e.g., dredging waste).
13.2.4. Deep-well injection
Simply put, very deep wells transport liquids to rock layers thought to lie underneath groundwater reservoirs. This once common strategy is still used to some extent, but it become more tightly regulated by federal laws enacted in the 1970s[1]. See Fig. 13.5.
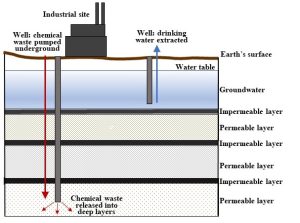
13.3. CURRENT PRACTICES
Changes to the size, lifestyles, and expectations of the human population during the past 100 or so years, combined with increasing understanding of the adverse consequences of mismanagement of waste, rendered practices used historically inappropriate in today’s world. We have learned that air, soil, and water are not infinitely able to dilute substances added to them. Indeed, by dumping, burning, and the like, humans have brought about appreciable changes to the chemical make up of Earth’s atmosphere and hydrosphere since the middle of the 19th Century. As we will see in Chapter 14, combustion of fuels and other materials has led to the accumulation of toxins in the atmosphere. Oceans, lakes, rivers, and other water reservoirs have similarly been polluted by various anthropogenic activities. Furthermore, a decreasing number of places on this planet are suitable locations for long-term storage of waste. In short, population growth and industrialization have led to increasing demand for resources and living space such that new approaches to waste management have become necessary. Here we explore some of the common modern strategies used to handle the three classes of anthropogenic waste defined above and meet the standards mandated by federal, state, and local laws (see Figure 13.6 for a summary of the fate of MSW in the U.S.).
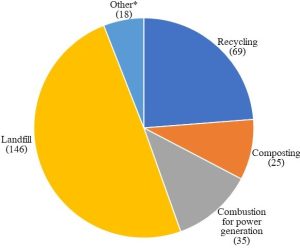
13.3.1. Municipal waste
Sanitary landfills
These underground storage sites isolate, or contain, certain types of low-toxicity trash such as that produced by households, office buildings, and schools. They employ a strategy that is functionally the opposite of the traditional dilution-is-the-solution-to-pollution approach because, rather than spreading waste out, landfills collect and concentrate it in relatively limited areas.
Landfill design and operation.
It is challenging to find appropriate sites
It likely goes without saying that few people get excited about the prospect of living near a landfill. Among the reasons for such reticence are concerns about the noise and congestion caused by large garbage trucks traveling on local roads, an increase in the number of scavengers (e.g., seagulls and turkey vultures) attracted to food waste, trash-related odors, aesthetic degradation of landscapes, and the decline of property values in affected areas. Furthermore, federal and state laws prohibit the construction of landfills in various places deemed inappropriate or particularly sensitive such as floodplains (Chapter 7) and wetlands (i.e., marshes, swamps, and the like). Companies seeking to open a landfill therefore encounter many political, economic, and legal barriers.
Large areas are divided into individual cells
Contrary to the belief held by some, landfills are not simply giant holes into which trash is dumped and buried. Instead, a large tract of land—generally several hundred acres—is divided into smaller subunits known as cells. Each cell is on the order of 10 – 20 acres in size and managed separately. Depth varies with location and can reach hundreds of meters or more.
Cells are lined with impermeable layers
According to federal law[2], modern landfills in the United States must be bounded on all sides by layers of clay and plastic to minimize the movement of water through garbage and the leaching of toxins to groundwater (Chapter 11). See Figure 13.7 for a diagram of a typical landfill cell. This practice is effective in restricting the movement of pollutants, but it creates some challenges (explained in “Disadvantages of landfills”, below).
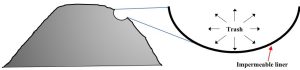
Materials are received and compressed to maximum density
A relatively small area within the landfill actively receives trash. At this location, known as the working face, trucks dump their contents. Large vehicles (weighing 40 – 50 tons) then repeatedly drive over the garbage to spread it into even and highly compressed layers (Figure 13.8).
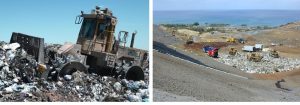
Cells are capped when full
The elevation to which a landfill may grow is dictated by the permit granted to the responsible company. In other words, a limited mass of trash is allowed before a site is considered filled. So, once waste has been compacted as much as is possible, the top of a cell is lined with additional clay and plastic to seal it on all sides indefinitely. Soil and grass seeds are applied to the surface above the cap (grass provides stability and resistance to erosion), and the site is then monitored for changes or breaches that could lead to environmental contamination (Figure 13.9). A new cell is opened and the process repeated until the entire landfill site has been filled to capacity.
Advantages of landfills.
Waste is contained
Modern landfills sequester unwanted trash and generally protect human and environmental health. Without them, unsightly and toxic materials could damage terrestrial and aquatic systems and pollute drinking water. Importantly, they use simple and mature technology and are relatively cost effective (compared to other methods discussed below).
Methane can be collected to generate electricity
Oxygen availability inside landfills is extremely limited. Consequently, organisms that can grow in the absence of O2 gas dominate these artificially created environments. Many anaerobic bacteria—particularly those thriving in landfills—release CH4, methane, as a product of their normal metabolism (review anaerobic and aerobic respiration in Chapter 4). Arguably, methane production is both a disadvantage and an advantage of landfills as a waste management strategy. It is problematic because this explosive gas could accumulate to dangerous levels within sealed cells if not removed by a vacuum system (Figure 13.9.a,b).
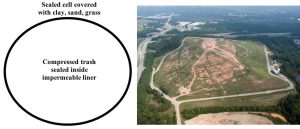
Historically, the collected gas was vented directly to the atmosphere, contributing to air pollution (Chapter 14), or burned at a large flare (Figure 13.10) (CO2, the dominant product of this combustion, is a pollutant that poses a lower risk than does CH4—more in Chapter 14).
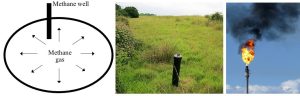
On the plus side, combustion of the methane can be used to produce electricity (consult Chapter 10 for more about electricity generation). This process, an example of what is often called waste-to-energy, has become increasingly popular during recent decades. According to the U.S. EPA, about 75% of active landfills in the United States now use captured methane in power generation[3].
Disadvantages and challenges of landfills.
Decomposition is very slow
Natural decomposition that would otherwise break down and recycle waste is severely limited in landfill cells because the aerobic microorganisms capable of efficiently carrying out this function are deprived of necessary oxygen. Sealing garbage in water- and air-tight chambers minimizes water pollution, but it also creates an anoxic environment (i.e., lacks available O2). So, much of the paper, food, yard waste, cotton, and other such materials tend to persist in landfills for decades or centuries. If you were to tunnel deep into an old landfill (yes, some scientists do this willingly) you would very likely find many readily degradable items, for instance, newspapers and partially eaten hotdogs discarded in the early 1900s in near-pristine condition. In other words, trash is stored, not eliminated, in landfills.
Long-term containment and monitoring are required
The operator of a landfill is legally obligated to maintain the site for many decades after it has ceased receiving trash and been sealed. In addition to the methane collection system described above, special wells are drilled to detect potential leaching of toxins to groundwater. Overall site integrity is also monitored to protect human and environmental health.
Land use options are limited
Landfills take up an enormous amount of space. Sealed landfills may appear to be solid grassy mountains, but their surfaces are not appropriate for housing, agriculture, and the like because they change shape and lose stability as trash undergoes slow decomposition, shifts, and settles. In addition, concerns about contamination of water reservoirs and exposure of humans and animals to harmful materials substantially limit future use. Various recreational and industrial facilities—as well as solar farms (Chapter 10) have been constructed on top of closed landfills, although this practice is not commonplace (Figure 13.11).
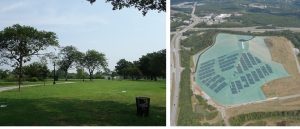
Incineration
In this second approach, waste is combusted to reduce its mass and volume. Contrary to the vision some people have, this is a complex process that is quite distinct from open burning (review above).
Incinerator design and operation.
Waste is combusted at high temperature
Municipal solid waste (MSW) is burned inside specialized facilities that resemble large, closed furnaces. Importantly, these incinerators operate at temperatures high enough to maximize destruction of trash (in the range of 1400 ºC or 2500 ºF, far higher than, say, a fireplace or oven[4]).
Ash is collected and landfilled
Combustion does not destroy trash, rather, it greatly reduces the volume of MSW. Results vary, but a decrease of 85 – 95% is typical[5]. The leftover ash must still be managed, so it is generally transported to a sanitary landfill as described in the previous section.
Toxic emissions are minimized
Heavy metals, dioxin, particulates, and other hazardous substances are produced when MSW is burned. Early incinerators were not equipped to control the release of such toxins, but modern incinerators employ mechanisms to largely prevent the movement of harmful products into Earth’s atmosphere.
Advantages of incineration.
Incineration reduces the need for landfill space
Incineration helps alleviate the problem of shrinking landfill availability because it dramatically reduces the volume of MSW needing to be managed.
Trash can be used as fuel to generate electricity
When MSW is burned, the heat released can be used to boil water, produce steam, and generate electricity (Chapter 10). Thus, solid waste incineration is another example of the waste-to-energy strategy described above.
Disadvantages and challenges of incineration.
Risk of air pollution
We saw earlier that the burning of solid waste produces many toxins. Since these substances threaten human and environmental health, public opposition to construction and operation of incinerators tends to be intense. It turns out that laws governing MSW combustion in the U.S. are rather strict, and new facilities use several chemical and physical methods to stay within regulatory limits. Accidental releases are unlikely, but not impossible. On the other hand, emissions of carbon dioxide and water vapor are not controlled. Although trash incineration puts relatively small amounts of it into the atmosphere, CO2 is of interest because of its role in climate change (Chapter 14).
Need for management of toxic solids
The incombustible ash left after incineration is generally sent to a landfill. Since it may contain highly concentrated toxins, it must be handled carefully.
High start-up costs
Incinerators are very expensive to build. According to the U.S. EPA, construction of a typical facility requires at least $100 million[6]. Revenue is generated from fees for services as well as electricity sold to power companies, however, recovery of the initial investment may take decades.
Recycling
Many products that are integral to modern life—things such as containers, books, magazines, clothing, electronic devices, vehicles, buildings, and so forth—are collected and reprocessed after they are no longer functional, and the materials that made them up are incorporated into new objects. This notion that resources can be salvaged and used again should remind you of our earlier discussions of the natural pathways that move materials within and among spheres of the Earth. Processes such as fixation, consumption, and decomposition drive the constant translocation, transformation, and repurposing of atoms throughout living and non-living reservoirs (Chapter 4). As noted earlier, materials do not accumulate in natural systems and are not otherwise discarded. Instead, they are recycled. Although the principle behind this waste management strategy is simple, the practice of synthetic recycling is rather complex.
Overview of the process.
Initial separation and collection of materials
You have likely seen the various containers intended to receive different waste materials: recyclable paper, glass, metals, and certain plastics are isolated from non-recyclables such as food, soiled paper, Styrofoam, and certain (other) plastics. Waste management companies collect the waste and haul it to the appropriate processing facilities. Trash that will not be recycled is transported to a landfill or incinerator, whereas the recyclables move on to the next step in a long journey.
Further separation of comingled recyclables
Materials must be separated from each other if they are to be efficiently recycled, and various strategies are used[7]. Typically, complex assembly lines made up of people picking objects off a conveyor belt, physical screens of varying sizes, and robotic devices separate papers, metals, plastics, and glasses from each other (Figures 13.12 and 13.13).
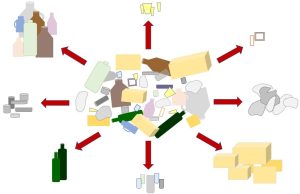
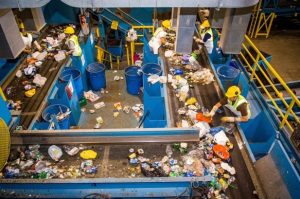
Each of those groups can then be further sorted into distinct, specialized streams. For example, magnets can isolate steel-containing cans from other metals. The many types of plastics (which correspond to the familiar numbers on the bottoms of soda, milk, yogurt, and detergent containers) are distinguished from one another by passing them in single file past a beam of light; since different plastics affect the light in different ways, an automated processor can push each object into a separate pile of like materials. Glasses of different colors may be similarly sorted by optical properties. Papers and boxes are handled by a combination of manual and other strategies. Sorting of comingled recyclables is a crucial step but is clearly quite time consuming and labor intensive.
Sorted recyclable materials can be sold, reprocessed, and reused
Individual groups of paper, plastic, and so forth are compressed and baled (Figure 13.14) so they can be easily transported and sold.
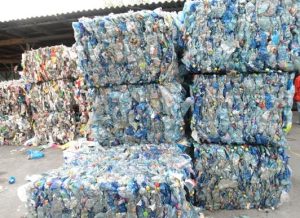
Like other commodities, a market of buyers and sellers influences their supply, demand, and price. These materials are used to manufacture a wide range of new products, but they are far from equally recyclable.
Glass. This is one of the few materials that we can reprocess and reuse an indefinite number of times with no appreciable degradation in its quality. Put another way, glass can repeatedly be melted down and serve the same function over and over.
Aluminum. Like glass, this metal is also highly reusable. Although some loss of quality does occur with each subsequent generation, it is fair to say that it can be made into new, functionally equivalent, products indefinitely. In fact, construction of new cans from recycled aluminum is easier and cheaper than it is from virgin material (the mineral bauxite) mined from the lithosphere.
Paper. Used paper is far less recyclable than glass or aluminum because its quality is substantially degraded with each generation: after six or so rounds, it simply can no longer maintain enough physical integrity to function adequately. Furthermore, the grade of products made from recycled paper diminishes with repeated recycling. Formal water-marked writing paper, for example, is generally made from fresh materials only. After this high-quality material is discarded, its reuse is limited to lower-grade newspaper and the like. Paper board and cardboard have similarly finite recyclability.
Textiles. Clothing and shoes can be recovered and reprocessed in different ways. Many of these items are cut up into rags or otherwise reused, but a very small fraction of the materials in textiles is converted to raw material to fabricate new articles[8].
Plastic. This is a more complicated story than that for other materials because the chemical and physical properties of different plastics vary so much (as noted above in the discussion of sorting). Although some can be reprocessed and used to construct new products like the old ones from which the materials were derived, most plastics cannot serve the same purpose more than one time. For instance, the plastic in food and drink containers is rarely made into new, equally usable bottles with equivalent properties, instead, it may be converted into unrelated items like jackets, furniture, and housing (Figure 13.15).
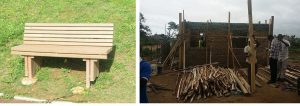
We will explore the challenges to effective plastic recycling in more detail shortly. In any case, research on plastics that can be better recycled is ongoing, and advancements could change the way plastic waste is managed.
Electronics. Mobile phones, computers, televisions, and related devices are built of multiple materials, including plastics, glass, and metals like lead, mercury, nickel, and zinc[9]. The last group—the metals—is particularly noteworthy. If recovered from electronic waste streams, these valuable materials can be sold to electronics manufacturers for reuse. Note, though, that collecting them from used devices is difficult and risky because many, like lead and mercury, are quite toxic to the humans that sort through them (Figure 13.16). We will return briefly to this topic near the end of the chapter.
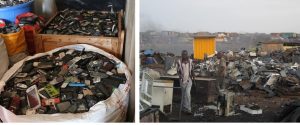
Advantages of recycling. Recycling provides many benefits. For example, materials that are recycled do not end up taking up space in landfills. The potential for pollution of soil, water, and air that can arise from mismanaged landfills and incineration is also reduced by recycling. Furthermore, recycling reduces the need to obtain new, or virgin, materials through processes such as mining (e.g., for aluminum) and logging (i.e., for paper). Rather than being discarded, materials can be reclaimed for future use.
Challenges of recycling.
Start-up costs and infrastructure
The processes described above require a sizable commitment from municipalities: money must be earmarked for construction, collection, transportation, separation, and maintenance of the many facilities needed. Although income can be generated from the sale of recycled materials, additional funding from taxes and grants is often needed to sustain recycling operations.
Contamination of waste streams
If you recall how the sorting strategies described above are intended to produce separate commodities that can be sold and recycled, you likely see the problem caused by contamination. Something like food waste in a batch of, say, recyclable paper, often renders the otherwise valuable material worthless. In such a case all the materials in a bundle would likely be landfilled or incinerated because the cost to clean the paper to an acceptable level is simply too high. In practical terms, educating and encouraging people to comply with labels like “Recyclables Only” is an important piece of this complex puzzle.
Manufacturing products so they can be recycled at the ends of their useful lives
Companies generally design, build, and market their goods with maximum utility and profitability in mind, but they do not always consider how to facilitate the recovery of materials that comprise them once these products cease to function. Among the ways recycling could be enhanced include simplified dismantling of complex objects like computers, cars, and the like and standardization of materials used within an industry. Furthermore, the problems of sorting and separation would be minimized if the number of different types of materials making up any manufactured product was reduced. Readers interested in learning more about overcoming design challenges should consult the books and articles written about these and related topics[10].
Final words on recycling. Before moving on to the final waste-management strategy on our list, let’s consider a few closing thoughts about recycling.
Reuse is not equivalent to recycling
Although these concepts do overlap, important differences between them should be noted. Simply put, if an object is repurposed without being broken apart and stripped of its raw materials, we will consider that to be reuse. So, refilling existing bottles, using old newspaper to line a birdcage, converting plastics or metal into artwork, altering existing clothing to produce new clothing, and even pretending a corrugated refrigerator box is a fort are all examples of reuse. On the other hand, when materials in existing objects are extensively reprocessed and used to construct completely new products, say when glass is melted and reformed into new jars, printer paper is shredded, pulped, and pressed into toilet paper, or plastic from two-liter bottles is chopped and reduced to pellets that are remolded into new containers, furniture, or clothing, then we use the term recycling. Of course, both strategies reduce the amount of waste entering landfills and incinerators, albeit in different ways.
Benches are not the same as bottles
We have already seen that not all materials are equally recyclable: some, like glass, can be reprocessed and reformed into new and equivalent objects whereas plastics (and some others) often cannot be used to construct objects that are the same as the source from which they were taken. The plastic recovered from beverage bottles, to recall an important example we noted previously, is generally used to make things such as park benches or warm-up jackets instead of products that serve the same purpose again. This practice of recycling materials into lower-quality goods is called downcycling by some because the materials are not able to accomplish the same task more than once. Importantly, downcycling necessitates large amounts of new raw materials to meet demand for high-grade containers and the like. Alternatively, converting used materials into products that are the same as, or even superior to, the original, is often termed upcycling. This later outcome more closely resembles natural biogeochemical cycles (Chapter 4) in which carbon and other atoms can be truly recycled without loss in their ability to perform the same tasks over and over and is, therefore, generally more desired than the former. Keep in mind, though, that upcycling often requires a very large energy input and may come with prohibitively high economic and environmental costs.
Materials intended for recycling may end up elsewhere
Objects made of recyclable materials can get mixed into waste streams heading for landfills or incinerators due to negligence, ignorance, expedience, or simple mistakes. These recyclables can also enter waterways or other natural systems, thereby damaging ecosystems and human health.
Despite recycling, Earth STILL is closed with respect to materials
Recycling and reuse can shrink the need for landfill space and extraction of new raw materials, however, they do not entirely overcome the constraints posed by the finite nature of Earth’s resources. These waste-management strategies certainly have an effect, but they alone will not shift us toward sustainability (Chapter 1). Arguably, for some people, recycling creates a perception of abundance and implies a license to use excess paper, plastic, glass, and so forth. The psychology of this phenomenon is clearly outside our study area, but it is context that the reader is encouraged to ponder. In any case, the need for less consumption—or, a lower rate of output in our familiar systems analysis model (Chapter 2)—will become ever-more urgent as standards of living and demands for goods and services rise worldwide. In short, there is good reason those three ‘R’ words, Reduce, Reuse, Recycle, are so often bound together.
Composting
We encountered composting in Chapter 9 when we studied agriculture (review Box 9.1 for more information). To remind you, natural processes are manipulated and harnessed to facilitate the rapid decomposition of plant remains from food, clothing, and paper into an organic-rich product that is very much like soil organic matter. It can be carried out in small, backyard bins or large, industrial-scale tanks that might be used by a restaurant, farm, or college dining hall (Figure 13.17).
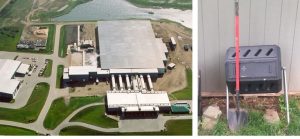
Bacteria and fungi, along with various detritovores (Chapter 5), quickly break down the scraps because the environmental conditions within composters are very favorable (e.g., high moisture, temperature, and nutrient availability). The product of this accelerated decomposition, compost, can then be applied to gardens and agricultural fields to increase soil fertility (Figure 13.18).
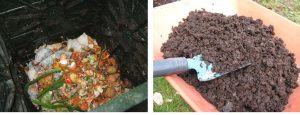
Composting brings benefits and challenges. On the upside, it reduces the amount of material going to landfills, a particularly important outcome because even food waste will not readily decompose in a sealed cell (as noted above). Furthermore, natural processes can be used to recycle nutrients to support crop growth in a relatively inexpensive manner (Chapter 9). There are challenges and disadvantages, though. Among other problems, compliance of users is needed, space is required, offensive odors may be released, and rotting food can attract unwanted rodents. Consult Box 13.1 to read about another potential problem with composting.
Box 13.1. Compost-ABLE vs. Compost-ED
Compostable bowls, forks, cups, and the like are becoming increasingly common. They may sound brilliant, but you should keep in mind that they will only break down as advertised if they are carefully collected and transferred to a composting facility. If, however, they are buried in a conventional landfill, they will persist like the food and similar materials we encountered back in the description of sanitary landfills in 13.3.1. In other words, compostable waste will only become compost under appropriate environmental conditions.
Methods of MSW management are typically used in tandem
No single strategy is appropriate to manage all the waste humans generate, so an approach known as Integrated Waste Management (IWM) is often employed. With IWM, a municipality develops a customized plan that combines two or more of the methods described in the previous section to meet its needs. One city might use a combination of incineration and recycling whereas another could rely more on landfills, composting, and recycling. In short, plans differ from place to place (and might even evolve with time), but most endeavor to minimize both financial and environmental cost.
13.3.2. Hazardous waste
Since these materials are highly toxic, they cannot be sent to MSW landfills or incinerators and are, instead, separated and managed in facilities especially designed for them. The guiding principles are the same here, though, as we saw for municipal waste: protect human and environmental health in the most cost-effective way possible. Several high-profile cases of environmental contamination in the 1970s led to strict federal laws in the United States regarding hazardous waste (review Chapter 1, including Table 1.1). The Resource Conservation and Recovery Act (RCRA, 1976 and later amendments), for example, mandates so-called cradle-to-grave management of hazardous waste, meaning producers, transporters, and disposal facilities are required to track every step of the management journey of regulated chemicals. Laws such as RCRA are intended to protect human and environmental health by establishing uniform standards of handling and record keeping so responsible parties are held accountable in the case of accidental releases (and, to avoid another case of abandoned chemicals such as the one in Love Canal described in Chapter 1). Here we briefly consider some of the common strategies used to manage these risky materials.
Hazardous Waste Landfills
Some hazardous waste end ups in secure landfills, structures that are conceptually like sanitary landfills in that they are intended to contain toxins and protect water, humans, and natural ecosystems. However, these structures are more complex than the ones we saw above, containing extensive monitoring systems to detect leaks, multiple layers of concrete and other strong materials (unlike the plastic liners used in MSW landfills), and more durable construction to maintain their structural integrity indefinitely.
Incineration
As with MSW, some hazardous waste is burned in facilities that are specially designed to operate at very high temperature and capture toxic emissions.
Recycling
Some solvents and other hazardous materials can be processed and recycled, thus reducing the amount of materials going to landfills and incinerators. As it can be difficult and expensive to separate recyclables from the rest, this option can only be used in certain situations.
13.3.3. Biomedical waste
Since human fluids and tissues (and the plastics, glasses, metals, and other solids contaminated with them) have the potential to spread infectious diseases, they are kept separate from municipal waste. Biomedical waste, like hazardous waste, is subject to careful monitoring, containment, and disposal. Generally, materials are first sterilized by heat or other means and then burned in specially designed incinerators. The resulting ash may be sent to municipal landfills if it is deemed free of contaminants or stored in a more secure site if it still contains hazardous substances.
13.4. CAN WE REDUCE WASTE?
It would be fair to say that waste minimization—the production of the smallest volume of unwanted products—it a goal of most stakeholders in the public and private sector. Some people take waste minimization a step further and seek to eliminate the need for landfills or incinerators. As its name suggests, the Zero Waste Movement aspires to a system in which 100% of our materials are recycled or reused indefinitely. Achieving its goal would necessitate adjustments to consumption habits and product design, as we considered above, and a transformation to a system modelled on Earth’s natural processes (refer to the opening page of this chapter). The challenges impeding a move to zero waste are numerous, however, such a system would alleviate many of the problems associated with waste generation we have already considered, including finite landfill space, air and water pollution, and loss of useable materials that are discarded.
13.5. CONSEQUENCES OF MISMANAGEMENT
As we have implied throughout this chapter, a lack of appropriate and effective waste management can cause water, air, and soil pollution. Also worrying is the potential for direct exposure of human and non-human organisms to poisonous materials in various waste streams (more in Chapter 15). Animals scavenging in open dumps, as well as people trying to recover valuable metals in electronic waste, are among those that can be adversely affected by poor management of discarded materials.
We will close this chapter with a few words about a serious threat that has gained prominence in recent years. Littering, accidental releases, and intentional dumping have introduced great quantities of physical pollutants into oceans and other bodies of water. Plastics are particularly important because they tend to resist decomposition and persist in waterways indefinitely. Large floating debris can entangle, suffocate, or otherwise hinder organisms that encounter it. In addition, very small fragments—known as microplastics—can cause damage to individuals, ecosystems, and humans (see Box 13.2 for more about this growing problem).
Box 13.2. Large ocean, tiny particles
Microplastics—defined as objects between 1 nm[11] and 5 mm in any dimension[12]—end up in the oceans for a couple of reasons. First, large bottles and related objects can undergo some degradation in the intense sun along coastlines and start to break up into small pieces that wash into water (Figure 13.19.a,b).
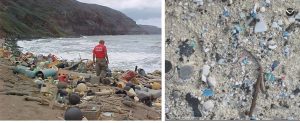
Second, small plastic beads intentionally added to some consumer products like toothpaste and cosmetics pass through the sewage treatment plants we encountered in Chapter 11 (Figure 13.20).
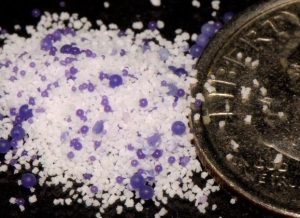
Although research on this phenomenon is ongoing, data suggest that these microplastics are often mistakenly seen as food by fish. So, what’s the big deal with eating tiny debris? Among other concerns, consumption of small particles can fill an animal’s gut with non-nutritive solids and lead to starvation. Furthermore, since some chemical pollutants (e.g., industrial and agricultural products) often bind to these plastics, exposure to toxins can increase in affected organisms. The adverse consequences experienced by an individual may then be passed to and magnified in consumers at higher trophic levels, including humans (review trophic interactions in Chapter 5)[13], [14], [15]. More about the movement of pollutants through food webs is presented in Chapter 15.
THE CHAPTER ESSENCE IN BRIEF [16]
Increasing population size, standards of living, and resource use have led to a growing need for effective waste management. Several adverse consequences result from a lack of appropriate measures, including the loss of discarded materials for future use, the filling of Earth’s finite space with trash, and the contamination and degradation of natural systems. Several strategies are used to minimize the problems of waste, but all bring both costs and benefits.
Think about it some more…[17]
Thinking back to our definitions from Chapter 7, what are the important differences between the fates of natural waste and those of waste produced by human activities?
Why is “disposal” a misleading word to use in the context of waste management? Think about our fundamental principles in environmental science (Chapter 1) as you ponder your answer.
Why is containment of waste such a critical concern (think back to what you learned about groundwater in Chapters 4 and 11)?
Thinking like an environmental scientist and remembering the ways environmental conditions influence organisms, why is decomposition in landfills so slow?
What strategies could we use to improve recycling so it better mimics decomposition and reuse in natural ecosystems?
If you were in charge of making the relevant decisions, what would you do to reduce our waste management problems?
- US Environmental Protection Agency (EPA). Protecting Underground Sources of Drinking Water from Underground Injection (UIC). https://www.epa.gov/uic/underground-injection-control-regulations-and-safe-drinking-water-act-provisions ↵
- US Environmental Protection Agency (EPA). Municipal Solid Waste Landfills. https://www.epa.gov/landfills/municipal-solid-waste-landfills ↵
- US Environmental Protection Agency (EPA). Basic Information about Landfill Gas. https://www.epa.gov/lmop/basic-information-about-landfill-gas ↵
- Mass.gov. Municipal Waste Combustors. https://www.mass.gov/guides/municipal-waste-combustors#-about-combustion-facilities- ↵
- US Environmental Protection Agency (EPA). Combustion with Energy Recovery. https://archive.epa.gov/epawaste/nonhaz/municipal/web/html/basic.html ↵
- US Environmental Protection Agency (EPA). Combustion with Energy Recovery. https://archive.epa.gov/epawaste/nonhaz/municipal/web/html/basic.html ↵
- US Environmental Protection Agency (EPA). Reduce, Reuse, Recycle. https://www.epa.gov/recycle ↵
- National Institute of Standards and Technology (NIST). 2022. Your Clothes can have an Afterlife. www.nist.gov/news-events/news/2022/05/your-clothes-can-have-afterlife ↵
- US Environmental Protection Agency (EPA). Electronic Waste and Demolition. https://www.epa.gov/large-scale-residential-demolition/electronic-waste-and-demolition ↵
- William McDonough has written much on these topics, including Cradle to Cradle: Remaking the Way We Make Things (McDonough and Braungart, 2008) and The Upcycle: Beyond Sustainability - Designing for Abundance (McDonough and Braungart, 2013). ↵
- nanometer, 0.000000001 meters ↵
- US EPA. Microplastics Research. epa.gov ↵
- What are Microplastics? National Oceanographic and Atmospheric Administration (NOAA). https://oceanservice.noaa.gov/facts/microplastics.html ↵
- US Environmental Protection Agency (EPA). State of the Science White Paper: Effects of Plastics Pollution on Aquatic Life and Aquatic-Dependent Wildlife. EPA-822-R-16-009 ↵
- www.epa.gov/trash-free-waters/state-science-white-paper-effects-plastics-pollution-aquatic-life-and-aquatic ↵
- As you will find throughout this book, here is very succinct summary of the major themes and conclusions of Chapter 13 distilled down to a few sentences and fit to be printed on a t-shirt or posted to social media. ↵
- These questions should not be viewed as an exhaustive review of this chapter; rather, they are intended to provide a starting point for you to contemplate and synthesize some important ideas you have learned so far. ↵
A law first passed in the U.S. in 1970, it was intended to reduce air pollution. Several subsequent amendments have strengthened it over the years. See Chapters 1 and 13 for more.
A large-scale approach to the clean up of human sewage. Sewage treatment plants are typically operated by counties, cities, or other governmental organizations. See Chapter 11, especially Figure 11.13, for more.
A gaseous form of C consisting of 4 H atoms bonded to 1 C atom. See also natural gas. See Chapters 4, 10, and 13 for more.
A general word that refers to the breaking down of relatively large, complex materials into relatively small, simple materials. Certain organisms, known as decomposers, break down the remains and waste products of other organisms and use those products as nutrient sources. Decomposers are critical to the health of ecosystems because they aid in the recycling of materials. Fungi and some bacteria are important decomposers. See Chapters 4 and 5 for more.
An adjective referring to an organism that uses dioxygen in its metabolism. "Aerobe" is the noun, the organism itself. Contrast with anaerobic. See Chapter 1 for more.
A reservoir of the hydrologic cycle located below the Earth's surface. See Chapter 4 for details.
Areas at which many solar panels are concentrated; electricity generated at such a site could be distributed to multiple users. See Chapter 10 for more.
A general term related to biogeochemical cycles. It refers to any process that increases the biological availability of an atom. See Chapter 4 for details.
A process whereby an organism ingests nutrients through diet. An organism that carries it out is a consumer. See Chapters 4 and 5 for details.
Movement of material from one place to another; often used to refer to movement among reservoirs. See Chapters 1 and 4 for more.
A chemistry term, atoms are the building blocks of everything in the universe. They are the smallest units into which an element can be divided. Atoms consist of even smaller, subatomic particles, such as protons, electrons, and neutrons. See Chapter 4 for more.
A practice that involves the accelerated decomposition of non-animal waste such as plant scraps, leaves, and related objects. Materials are collected in a structure known as a composter in which environmental conditions are maintained to stimulate aerobic decomposition. Compost, the product, is an organic- and nutrient-rich material that can be used as fertilizer. See Chapters 9 and 13 for more.
Refers to the many different kinds of microscopic organisms that are single celled and prokaryotic (i.e., do not possess distinct cellular organelles). Collectively, bacteria are extremely diverse, are the oldest organisms on Earth, and are critical to many environmental cycles and processes. See Chapter 3 for more.
Classed as eukaryotic microorganisms. Some are unicellular (e.g., yeast), many are multicellular (e.g., mushroom). They are are heterotrophic and tend to act as important decomposers in ecosystems. See Chapter 3 for more.
Small organisms that feed on the decaying remains of other organisms. See Chapter 5 for details.
City in western New York State (USA) that became famous when hazardous waste buried beneath it came to the surface. National attention and outrage led to new environmental protection laws. See Chapter 1, especially Table 1.1, for more.